CFD Sheds Light on Beirut Explosion
Blast simulations provide greater insight into causes of the tragedy, and how the explosion affected surrounding structures.
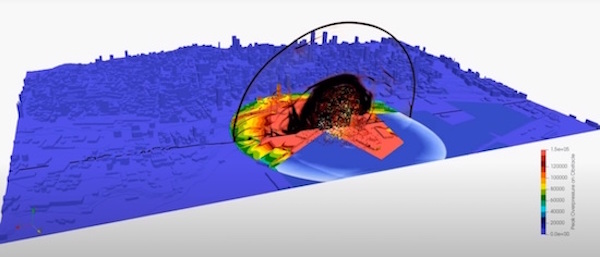
The Lebanon blast simulation in Viper::Blast. Image courtesy of SSSL.
Latest News
September 28, 2020
On August 4, 2020, the world witnessed two sequential explosions in the city of Beirut, capital of Lebanon, which caused immense destruction to the city, and left 200 dead and 6,000 injured (as of today).
The first explosion was apparently the result of stored fireworks igniting. The second, more destructive explosion was the result of the firework explosion and fire detonating a store of impounded ammonium nitrate, which had previously been confiscated from a ship, the MV Rhosus.
The MV Rhosus had attempted to sail from Georgia to Mozambique in 2013, but upon arrival in Beirut, the ship was deemed to be unseaworthy. In 2014, the cargo of 2,750 tons of ammonium nitrate (which was destined for the mining industry in Africa) was brought ashore and stored in Warehouse 12 in the Beirut port, where it sat, largely forgotten, until the explosion.
The detonation of these explosives left witnesses on the ground and online stunned as the fireball and shockwave devastated the city.
In the immediate days that followed, images and videos of computer simulations of the blast reached social media, compelling journalists to ask questions about the nature and purpose of the simulations.
We also reached out to the company behind the simulation software to find out how computational fluid dynamics has been used for blast modeling in the case of this incident.
Weapons Effects Simulator
We reached out to Scotland-based CFD explosive simulation company, SSSL, which has developed a CFD blast-modeling software called Viper::Blast.
In fact, the company website describes the software specifically as a “weapon effects simulator.”
We spoke to Will Wholey of Arup, and Christopher Stirling, who founded the company in 2017, about how their Viper::Blast software has been used in the aftermath of the explosion.
“We understood that our blast analysis skills and powerful software enable us to quickly offer a more technical perspective on the blast than was being offered by the discussion that was taking place at the time on social media,” said Wholey.
“We wanted to understand for ourselves how the blast wave may have interacted with the complex terrain and buildings around the harbor and the city. It was out of our own interest and initiative rather than any external commission that we joined up to see what we might be able to model and understand in a short amount of time,” he said. “Each member of our team brought a different set of skills and knowledge to the task.”
Analysis Team
The aforementioned team comprises Stirling himself, and members of Arup’s Resilience, Security & Risk group (RS&R), namely Julia Abboud, Wholey and also Ian Bruce (from the Arup Advanced Technology & Research team).
“Arup and SSSL worked together on processing the geometry and assembling the model for the blast analysis,” said Stirling. “Both SSSL and Arup ran similar models with the same geometry and data (charge size), however, we each ran them on our own hardware.”
Quick Turnaround
Any simulation engineers who have already seen the simulation plots may wonder how the team managed to produce their work in such a short period of time. The plots themselves appeared on social media within a day or so of the incident. That is a very quick turnaround for a complicated CFD simulation.
We asked SSSL how they managed to produce the simulations so quickly, and what data they had access to. After all, modeling entire city blocks, meshing and running CFD simulations can be tedious and slow at the best of times.
“The only data required in order to undertake our simulation is geometry, charge material (reported widely in the press), and charge size (also reported widely in the press),” said Stirling. “We found the geometry via Google (quickly). The charge size and material were reported on approximately 24 hours after the event. We started our analysis once these things were known.”
“Geometry was available online in the format of a Rhino model, listed as ‘provided to the public,' with credit to Salim Al-Kadi (architect) and his team,” explained Stirling.
“For the charge size, we used the widely reported figure of 2,750T of ammonium nitrate from various news sources. We “offer no judgment on whether this figure is perfectly accurate for the blast source; it was a credible number to get started with our analysis,” Stirling said. “We were able to undertake the modeling so quickly because of the high quality of the model geometry that we found online—it didn’t require any modification or substantial cleaning—and because of the incredible speed of the CFD software.”
Fast Simulation
Generally, complex CFD simulations require a lot of computational power to reduce wall-time of calculations. However, the Viper::Blast software does not necessarily require the entire might of a HPC cluster as explained as follows.
“Viper::Blast is accelerated to run the analysis on GPUs rather than on clusters of CPUs, which makes it up to hundreds of times faster (for the same resolution / level of detail) than similar CPU-based methods,” explained Stirling. All this is possible on hardware that can be bought for a gaming PC. It has changed our expectations of what is possible for blast CFD analysis.”
Arup’s model took 4.75 hours on a high-end gaming PC, an Alienware Area-51 R5, and SSSL made use of the Google Cloud Compute Virtual Machine, resulting in a runtime of around 5 hours.
Data Inputs and Outputs
As we have heard already, the simulations were enabled thanks to readily available CAD models online combined with data widely published in the media (namely information on explosive materials and yield).
Within hours of receiving the information, SSSL and Arup were able to produce the simulation plots and videos you see in this article.
But what do these plots show?
“Typical outputs are of absolute pressure, density, energy, peak overpressure and peak impulse,” said Stirling.
“These metrics are primary fluid field (air mainly) related quantities and all vary with time as a blast propagation develops. Other more complex metrics such as probabilistic risk of injury, primary blast injury (lung damage, ear drum rupture) can also be output,” he said.
With the data visualizations provided by the simulations, SSSL and Arup hope to spread awareness about the storage of ammonium nitrate, and to provide an enhanced technical narrative of what occurred on the day.
The information helps to show how the blast wave interacted with structures in the area, and also how much energy was involved in the explosion (how much explosive material actually detonated). This could be confirmed by back-correlating damage models with physical investigations of the damage on the ground.
Eventually, the simulations could help shed light on how buildings perform structurally in such events, and will perhaps inform construction companies and urban planners on what is an acceptable hazard when designing for infrastructure resilience.
Conclusion
Aside from the technical goals of using Viper::Blast, there is a strong outreach element as to why the team took on this project.
“That is part of our aim in publishing our post; we want to express our great sympathy and concern for Beirut, and hopefully raise awareness of the great needs of the people of Lebanon right now,” said Stirling.
“Further into the future, and in a more technical setting, the results of similar modeling could be used to better characterize and investigate the true amount of ammonium nitrate that detonated and enhance our understanding of various established practices for hazard mitigation of ammonium nitrate storage,” he said. “Perhaps studies similar to this one will assist in preventing a disaster of this sort from happening again by showing that this kind of preventable tragedy can be analyzed, and resources allocated to alternative, safe, appropriate storage of ammonium nitrate.”
Sources: Press materials received from the company and additional information gleaned from the company’s website.
Subscribe to our FREE magazine,
FREE email newsletters or both!Latest News
About the Author
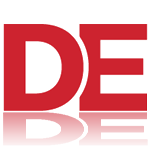
DE’s editors contribute news and new product announcements to Digital Engineering.
Press releases may be sent to them via [email protected].
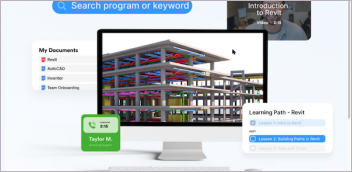
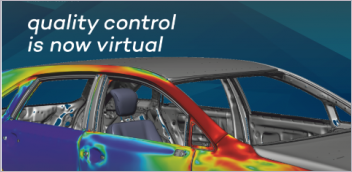
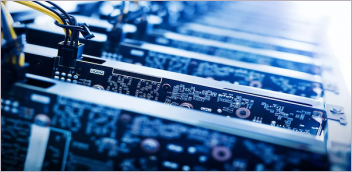
