A Deeper Look into Metal Additive Manufacturing Material Properties
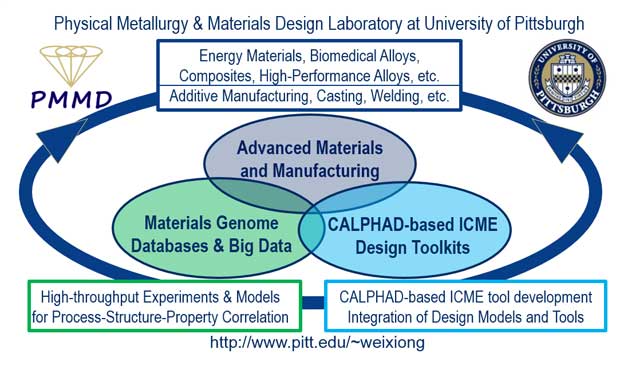
Figure 1. Researchers at the University of Pittsburgh have combined CALPHAD-based ICME design and simulation tools with materials genome databases to enable engineers to simulate the entire additive manufacturing process. This simulation provides new insight into the impact of AM processes on the microstructure and material properties of parts produced for high-temperature applications. Armed with this new capability, engineers and manufacturers will be able to certify the quality of AM-produced parts. (Image courtesy of Professor Wei Xiong, Physical Metallurgy & Materials Design Laboratory, University of Pittsburgh.)
Latest News
March 30, 2017
Researchers at the University of Pittsburgh have been working with ANSYS to create a simulation technique that can evaluate the effects of additive manufacturing (AM) on the microstructure and material properties of parts produced for high-temperature applications. Up to this point, the only way to certify the quality of these parts has been to perform comprehensive physical tests. Unfortunately, these procedures have proven to be too costly and time-consuming.
The Crux of the Matter
Design engineers find themselves in this predicament partly because of the relatively rapid evolution of AM technology. Engineering firms originally used the technology to make plastic models and prototypes for prospective applications. Use of the technology, however, has expanded. Most recently, manufacturers have begun to employ AM to produce sensors, brackets and ventilation ducts. While these products hold value, a whole class of applications remains beyond the reach of most engineers and manufacturers. The drive now is to find ways to not only produce more types of parts using metal AM, but also to certify the quality of the parts in a cost-effective manner.Enhancing AM production and quality control in this way promises to open the door to greater flexibility for design teams and improved efficiencies for manufacturers. Traditional manufacturing often must combine smaller components to make subsystems because fabrication facilities are limited by the shapes that they can mold or mill. Using metal AM, manufacturers can build subsystems in layers, reducing the part count to a few components. This in turn will reduce the materials, fabrication processes and labor required to produce the subsystems.
The Catch: Certifying Material Properties
The problem confronting manufacturers isn’t that they cannot produce metal parts for high-temperature applications. The catch is that the aerospace industry and other sectors require manufacturers to certify the strength, flexibility and consistency of the parts they produce.What manufacturers need is a quick and inexpensive way of determining how the interactions between the laser or electron beam and the metal powder used in the AM process affect the part’s final state. Manufacturers must also be able to determine if microstructure variations can occur from one part to another. Because the metal melting process is so fast, designers and manufacturers find it difficult to quantitatively measure the thermal profile of the alloys. The heating process also affects the microstructure’s evolution, including internal stress of the materials.
The challenge here is the complexity of the AM processes.
“There are so many variables with uncertainties in materials processing, especially in the metals 3D printing process,” says Professor Wei Xiong, one of the leading members of the university’s research team. “Without predictive simulation, it is hard to control the material’s quality. High-temperature materials used for jet engines are usually critical components, requiring ultra-high mechanical performance. Therefore, it is important to perform reliable simulation to optimize processing parameters for the best quality of the 3D-printed components.”
Thus, before AM can take on more demanding applications — such as broader reliance on short runs of certified parts — designers and manufacturers need to better understand the impact that AM has on the quality of parts.
A Window on High-Temperature AM
To meet this requirement, the researchers at the university’s Department of Mechanical Engineering and Materials Science have been perfecting a simulation process that they hope will ultimately visualize the impact of AM, focusing on features like strength and creep. To this end, the team developed an integrated phase transformation and grain texture model to predict microstructure evolution under metal AM’s heating and cooling cycles. Such a model promises to reduce or eliminate the need for costly and time-consuming physical testing and opens the door for a simulation-based certification for AM parts that will satisfy demanding industries.To build this model, the researchers opted to base the simulations on Integrated Computational Materials Engineering (ICME), which allows engineers to design products by linking materials models at multiple length scales (Figure 1). This approach aims to provide greater insight into how various fabrication processes produce material structures, how the structures generate material properties, and how engineers should choose materials and tailor processing parameters for a given application.

“ICME is a landmark method for advancing computational materials modeling in practical engineering applications,” says Xiong. “The ICME tools will enable us to predict alloy microstructure [produced] by different processing, and thus further control material properties.”
Moving Forward
The ICME techniques and tools developed by the researchers at the University of Pittsburgh are still in the development stage, but the technology promises to open a raft of new applications for AM. In the process, the simulation technique could well make high-temperature metal AM accessible to a broader spectrum of manufacturers. Armed with this technology, engineers will be able to use the simulation and design tools to fine-tune AM processes and gain insights into how they can modify operating parameters to achieve specific results.Success of these simulation techniques and tools will translate into a broader role for AM in the industrial sector, sweetening the deal by shortening development cycles. Without simulation tools such as these, high-temperature metal AM could remain a costly specialty technology beyond the reach of most design teams and manufacturers.
Subscribe to our FREE magazine,
FREE email newsletters or both!Latest News
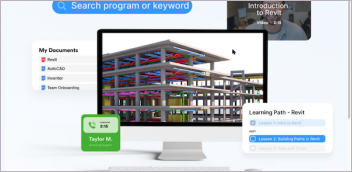
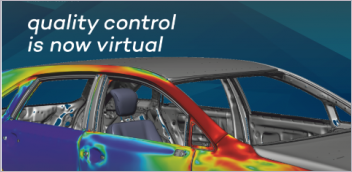
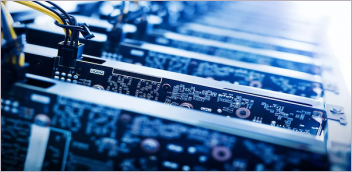
