Latest News
April 1, 2010
By Susan Smith
On August 25, 2009, NASA took six materials experiments into space aboard the space shuttle Discovery in its first Materials Science Research Rack (MSRR-1). These experiments were conducted onboard the shuttle by astronauts and integrated into the U.S. Laboratory Module Destiny, the primary research laboratory of U.S. payloads, on the International Space Station.
![]() European Space Agency Astronaut Christer Fuglesang (left background) and NASA Astronaut Tim Kopra, both STS-128 mission specialists, install a Materials Science Research Rack-1 (MSRR-1) in the Destiny laboratory of the International Space Station. |
The MSRR-1, developed by the Marshall Space Flight Center, in Huntsville, AL, is part of a collaborative research program with the European Space Agency (ESA). The MSRR-1 carries the ESA-Materials Science Laboratory Low Gradient Furnace (MSL-LGF) to be used for future low-gravity materials-science experiments by astronauts. Two of the experiments are U.S. projects and four are European.
The two U.S. experiments focus on the ways single-crystal castings solidify on earth vs. in space. As critical components in high-temperature gas turbine engines used in high-speed aircraft and land-based power turbines, single-crystal castings are subject to convection, the transfer of heat by movement. Because of this, they frequently end up with defects (misaligned grains and macro segregation) and can’t be used for applications such as airplane engine blades.Space has less convection, so researchers are interested in finding out if and how single-crystal castings solidify differently in space. The theory is that single-crystal castings solidify in space free of convection-related defects. If this is true, it would have widespread ramifications for industry.
What Constitutes the MSRR-1
The MSRR-1 is comprised of a thermos called a Materials Science Laboratory in a refrigerator-sized body developed by the ESA. Within the Materials Science Laboratory is the MSL-LGF that was transferred from the shuttle onto the Space Station.
“There are currently 13 experiments planned, these are all materials-science experiments,” said Surrendra Tewari from Cleveland State University, head of a team responsible for the U.S. experiments. Of those 13, six are part of this current flight.
![]() Top left: a directionally solidified alloy cylinder (9mm diameter and 29cm long). Bottom left: an ESA sample cartridge assembly. At right: a single crystal Al-7% Si alloy dendrite array. |
Tewari said each sample is approximately 9 millimeters in diameter and about 10 inches long, cylinders of a 7 percent silicon aluminum alloy. The cylinder is kept in an alumina crucible, which in turn is encapsulated in a tantalum closed-end cylinder (as protection) from which electrical wires and thermocouples, etc., protrude. Each assembled piece is called a Specimen Cartridge Assembly (SCA).
For each one of these 13 experiments, there is one cartridge assembly per sample. “The astronaut will open this thermos door, which looks like a pressure cooker which is sitting horizontal, and insert this one cartridge into the cylindrical furnace,” says Tewari. “Then they’ll have a laptop, which will already have a preprogrammed temperature profile loaded into it. They’ll turn it on and the furnace will begin to process the samples. This will happen with each one of these samples.”
Low-Gravity Directional Solidification
Tewari described the process thus: To cast a turbine blade for an airplane engine or a power turbine, you usually create a ceramic mold of the blade into which is poured molten metal that is going to solidify at many locations. Each part that solidifies independently is a grain or crystal. In the usual casting process, many such grains or crystals are formed. If, however, you allow only one grain of solid to form at one end of the cavity and enable this one grain to grow, the solid that results will be a single grain or a single crystal. This is achieved by “directional solidification,” or freezing in one particular direction.
There is a liquid at the top, solid at the bottom, and a mushy zone in between where solid and liquid exist together. As you pull the mold away from the hot zone of the furnace toward its cold zone, the mushy zone is continually forming and solidifying, finally yielding to the directionally solidified casting.
The mush consists of long slender tree-like branched fingers of solid called dendrites. In between the dendrites is the melted liquid. When there is fluid flow (convection) in the melt, then these dendrites break, change directions, form new grains, and these in turn show up as defects in the final casting. How the dendrites are arranged is critical, says Tewari. When making turbine-blade castings on earth there is always convection because of gravity. As soon as one end of the fluid becomes hot and the other is cold, the fluid begins to flow.Computational fluid dynamics (CFD) professionals have tried to model that. Yet the spacing between the dendrites is of the order of 100-150 micrometers, whereas the sample size in this case is 9 mm, and the actual turbine blade is several inches in diameter. So the fluid flow is occurring over a scale that is millimeter to centimeters, but the solid that is forming is at a scale of several micrometers. Therefore, at this stage the fluid dynamics models are not capable of being able to predict what is happening in the solid at the micro scale. Defects are formed like disorder in the dendrites, nucleation of new grains that are totally misaligned in a different direction altogether.
“What we’re trying to do is to see if these defects can be completely eliminated when we don’t have fluid flow present in space,” says Tewari, “because in space there is no gravity or very little gravity, therefore we’ll expect there will be no flow, hence some of these defects that we observe will not be observed in space. So that’s one purpose. The second purpose is to quantitatively compare predictions from the current theoretical models with the experimentally observed size and distribution of these dendrites. The experimental data we’re generating on Earth always has convection associated with it, but the theoretical models that we are using to compare the data with have got only diffusion and no convection….”
To Outer Space and Back
ALCOA partnered with Cleveland State University to provide the aluminum silicon alloy bars. These were re-directionally solidified and converted into directionally solidified single-crystal dendritic seed bars at Cleveland State. Then the bars were sent to ESA in France where they were machined and assembled into SCAs. The rods were expected to return to Kennedy Space Center via the return of the STS flight 20A in February 2010.
When the samples return, scientists will cut them up and look at the microstructure as it changes along their length. The next sample will return about eight or nine months after the first one.
More Info:
ALCOA
Contributing Editor Susan Smith is DE’s expert in rapid technologies and has been immersed in the tech industry for more than 17 years. Send e-mail about this article to [email protected].
Subscribe to our FREE magazine,
FREE email newsletters or both!Latest News
About the Author
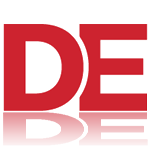
DE’s editors contribute news and new product announcements to Digital Engineering.
Press releases may be sent to them via [email protected].
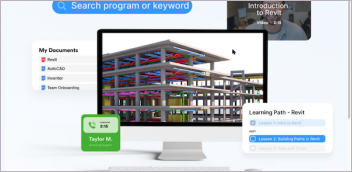
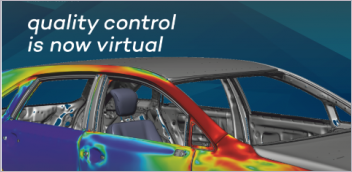
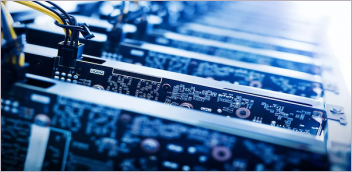
