Prescribing a Healthy Dose of DfAM
Thanks to maturing DfAM practices, companies can optimize and innovate medical device designs while meeting rigorous compliance requirements.
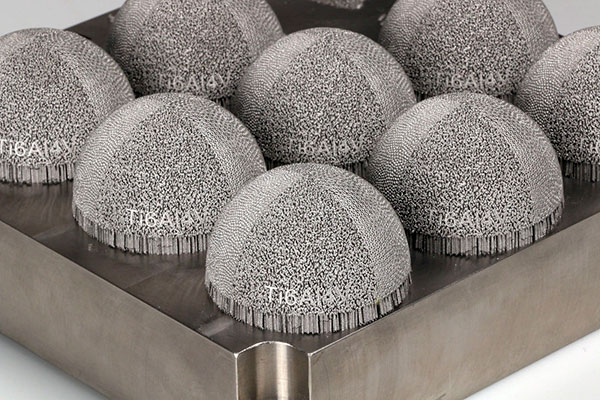
3D Systems has manufactured more than 2 million medical devices like these acetabular hip cups using DfAM practices and its FDA-registered ISO 13485-certified facilities in North America and Europe. Image courtesy of 3D Systems.
Latest News
March 7, 2024
As additive manufacturing (AM) gains traction for medical device applications, companies are struggling to mature design for AM (DfAM) practices to address long-standing regulatory requirements while facilitating the next wave of high-quality, patient-specific devices.
Companies have been leveraging AM to build medical device prototypes for decades, but the industry’s existing regulatory framework has remained mostly calibrated to conventional manufacturing methods without fully addressing unique characteristics of 3D printing.
The recent uptick in adoption of metal AM for production-grade applications, coupled with hospitals’ soaring interest in the Point of Care AM production model, has prompted a flurry of activity from the U.S. Food and Drug Administration (FDA). They, along with regulatory and standards bodies around the globe, have been engaging industry players to evolve guidelines and update policies and procedures to ensure FDA compliance for emerging 3D-printed medical-grade devices.
The Class III category, in particular, is garnering a lot of attention as devices like pacemakers, cochlear implants, and most notably, implantable prosthetics, are strong candidates for AM as a way to optimize and produce patient-specific designs.
As part of its concentrated push, the FDA issued a guidance document in December 2017 that detailed technical considerations for AM medical devices covering the full spectrum, including design and manufacturing processes, patient-matched device issues, materials controls and reuse, post-processing, process validation and acceptance as well as device testing.
In 2021, the FDA followed up with a revised framework outlining three manufacturing scenarios for 3D printed devices at the point of care. Last June, the FDA released additional draft guidance on patient-matched surgical guides for orthopedic implants, reaching out to the broader medical and AM community for their input.
“The regulatory piece has come so far so quickly,” says Scott Drikakis, medical segment leader at Stratasys. “At the end of the day, AM is just another manufacturing method so as long as companies follow outlined processes and procedures depending on whether they are building a Class 1, 2 or 3 medical device, it’s fine.”
While the regulations themselves aren’t necessarily a gating factor, organizations’ understanding of how to design specifically for AM to meet long-standing compliance standards is where maturity lags.
“Repeatability and the economics of AM have come a long way, but it’s still nowhere near where traditional manufacturing is when it comes to risk and redundancy,” Drikakis says. “That’s where there are gaps, and design for AM is a critical part of that story. I just don’t think people know how to do it.”
The DfAM Agenda
Manufacturers and product development engineers are accustomed to working the ins and outs of design for manufacturing (DfM) when it comes to conventional production practices like milling or injection molding, but the constraints and parameters are very different in an AM scenario. Too few engineers understand how to iterate designs based on specific AM requirements like how to create overhangs, incorporate supports and properly orient a build plate—all design choices that have even higher stakes when the end product is targeted for the human body.
“Understanding how AM is different than subtractive manufacturing is a key piece of what’s required,” Drikakis continues. “You can’t apply what you do for DfM to DfAM.”
FDA requirements surrounding cleanability and biocompatibility are factors that must be considered as part of the DfAM equation for medical devices. Those requirements can have an impact on materials selection, and there are already a limited number of FDA-compliant choices when you compare AM to subtractive manufacturing methods.
Well-established DfAM practices will also help engineers balance the trade-offs between a bespoke medical device design that incorporates organic shapes and complex internal cavities with the realities that any residual powder left in the body can endanger patient safety. With a DfAM-friendly approach, engineers are better equipped to zero in on a design that ensures toxic particles aren’t released into a body upon implantation, explains Ben Tuts, quality & regulatory affairs director at Materialise.
“People get started without a proper understanding of the regulatory and safety requirements,” Tuts says. “They optimize the design focused on manufacturing, but don’t necessarily optimize for the safety of the patient.”
Another issue is that existing FDA regulations haven’t been well adapted to the new design realities made possible with AM. For example, there are well-established strength requirements for medical devices like implants, which are applicable for both AM- and traditionally machined devices. However, those early predicate devices are a wholly different breed than an acetabular hip cup implant spun out of DfAM practices, which might include porous structures designed to foster bone growth to aid in strength and stability.
“That is the power of design freedom that 3D printing and DfAM offers,” says Gautam Gupta, senior vice president and general manager of medical devices at 3D Systems. “In some ways, AM enables innovation by delivering the in-growth requirements you want, but on the flip side, AM limits how much you can reduce the size of the inner core of the implant because the AM material is not as strong as raw material for conventional manufacturing. It’s all about finding the right balance.”
The validation process for mechanical structures is also different, and sometimes more challenging, with an AM-built medical device compared to one created with traditional machining, according to Gupta. Because there are so many factors that play a role in quality of output, engineers need to have a lot of intimate and detailed knowledge of AM processes and equipment.
“There are so many things going on that impact validation of a particular application—everything from laser power, powder size and layer thickness,” Gupta says. “Whereas machining is machining if you move from metal to plastic, if you change an AM technology or material, you have to validate” everything from scratch, he says.
Traditional metrology tools present another challenge as they aren’t cut out to analyze and inspect for limitations on the internal features that are so common with DfAM designs, explains Matt Shomper, an engineer who’s developed orthopedic implants for 15 years, specializing in using AM and computational design tools as part of the DfAM process.
It’s also difficult to prove a geometry will print consistently on every type of AM technology, he adds. “There’s no real way other than testing coupons for fatigue to prove that the AM process isn’t shifting over time,” Shomper says.
The range of variability when building patient-specific devices is another nuance that medical device makers need to take into account when planning regulatory and DfAM strategies. Scott Hollister, Ph.D., a professor at the Wallace A. Coulter Department of Biomedical Engineering at The Georgia Institute of Technology and the director of the tissue engineering & mechanics laboratory at Georgia Tech and Emory University, is well acquainted with those challenges as he moves through the process of getting FDA approval for a 3D printed tracheal splint implant intended for pediatrics applications.
While the implant has a fairly consistent shape, the size varies based on the patient, which could impact the ability to meet FDA requirements for mechanical properties. “You have to think about the potential range of sizes for patients based on age and gender, which can be difficult if there’s significant variability vs. always building to a standard set of sizes,” Hollister explains.
The ability of a device to degrade in the body over time and be excreted adds another dimension to DfAM that is unique to the medical device community. “If you’re designing to take advantage of AM’s ability to achieve complicated porosity or expecting materials to degrade over time, it’s a whole new level of criteria to design for,” he says.
Empowering DfAM
Thankfully, there are a variety of new technologies, tools and services that can help medical device makers advance their DfAM prowess and better navigate the still-evolving regulatory landscape. Advanced computational and simulation tools are essential for evaluating the complex design trade-offs that are part and parcel of successful DfAM strategies. Emerging 3D printer process monitoring technology can play a role in analyzing 3D builds and layer-by-layer powder melting to ensure parts are printed with the intended characteristics and within the specified quality parameters, Shomper says.
The first step is to immerse in existing guidance documents and examine any predicate devices that exist in the market, internally and from the competition. From there, it’s important to map a DfAM strategy that allows for maximizing critical features by leveraging the unique capabilities of AM, including looking at the entire workflow through the post-processing stages, not just at production, 3D Systems’ Gupta says.
3D Systems’ Application Innovation Group will consult with medical device makers at each step of the DfAM and AM journey, including how to make adjustments to designs to improve upon existing predicate devices along with how to qualify devices so they comply with industry standards and quality management services (QMS) practices.
For its part, Materialise also does regulatory consulting and maintains a deep bench of in-house DfAM experts that can guide medical device manufacturers through the entire lifecycle, from design to AM production through testing and validation. Materialise’s software solutions help accelerate DfAM practices, including the 3-matic design optimization software used to enhance models for 3D printing with textures, lattice structures and conformal structures; and Magics, a data and build preparation toolbox to optimize the AM workflow.
Point-of-care 3D printing is another area where Materialise sees significant growth and where it can lend support with DfAM and regulatory compliance. To support hospitals on this front, Materialise recently introduced the Mimics Flow Case Management suite, which helps hospitals labs track 3D printing and planning workflows to ensure quality and compliance and has inked a partnership with Ricoh USA, which will leverage Materialise software as part of its RICOH 3D for Healthcare, a HIPAA-compliant, ISO 13485 certified 3D medical manufacturing center used to make 3D printed anatomical models.
As AM gains a level of acceptability among medical device makers, it bumps up against the reality that comes with greater industry expectations. “AM comes with the vision that you can do anything,” says Andrei Pissarenko, Materialise’s R&D manager for medical. “Managing expectations from users while still providing a product that fulfills a need, meets expectations, and is safe and effective for patients; that’s the challenge.”
More 3D Systems Coverage
More Materialise Coverage
More Stratasys Coverage
Subscribe to our FREE magazine,
FREE email newsletters or both!Latest News
About the Author
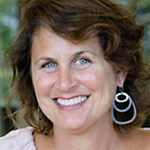
Beth Stackpole is a contributing editor to Digital Engineering. Send e-mail about this article to [email protected].
Follow DE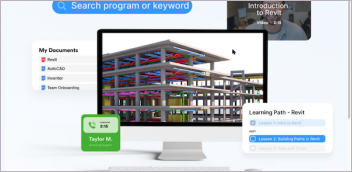
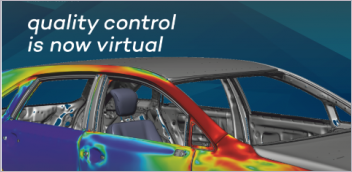
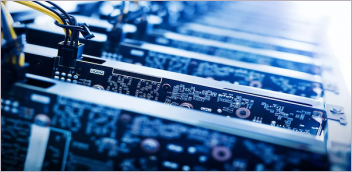
