Modeling Commercial Aquaculture Systems Employing FLOW-3D
Marine flow analysis using CFD is increasing shellfish production and minimizing its environmental impacts.
Latest News
November 1, 2009
By Pamela J. Waterman
Many factors influence marine aquaculture: tidal fluctuations, water temperature, food concentration, and stocking density, among others. Success in this industry depends on balancing what you can control with what you can’t, and putting years of experience behind day-to-day decisions. Nowadays there’s another tool to help identify productive sites and plan management strategies, especially considering sustainability: computer modeling targeted to aquaculture. A Maine-based business has been busy promoting this tool as an integral part of the shellfish aquaculture industry, and to prove it they use it to run their own commercial mussel farm.
![]() This shot of Killary Harbour, Ireland, shows longlines used for mussel harvesting. A Maine-based business has been using CFD to optimize longline placement. Images courtesy Blue Hill Hydraulics |
Challenges in Managing Water Resources
Since 2004 Blue Hill Hydraulics of Blue Hill, ME, has been using advanced numerical modeling methods to solve difficult flow problems. Their work in microfluidics, aquaculture, and river hydraulics has helped clients involved in dam removals, fish passage protection, and the design of laboratory equipment.
When the Irish Shellfish Association (ISA) approached Blue Hill Hydraulics (BHH) in 2005, they, like others, were looking for new approaches to improve the siting and operation of nearshore aquaculture operations. To increase the production of shellfish such as blue mussels, manila clams, geoducks, and oysters, BHH regularly answers questions about the number of shellfish that can be put in a particular area (i.e., optimum stocking density) and the best spacing to grow them to market size in a given period of time (i.e., 18 months).
![]() Mussel fishermen are using CFD to determine how best to arrange their longlines. |
Detailed answers to these questions depend on the specific type of aquaculture method used. Common techniques for growing mussels involve the use of rafts or longlines as the base structures from which to hang ropes suspended in the water. Farmers place seed mussels inside biodegradable “socks” that surround the ropes, then the mussels attach themselves to the ropes in a natural anchoring response that takes place before the socks decay.
With this method, planning factors include the length and diameter of rope, the design of protective predator nets, particulate (food) density in the ocean water, and water temperature. Similar issues are involved for oysters raised in flat net-bags stretched across gridded trestles. To reach the goal of maximizing the number of shellfish grown to market size in a minimum amount of time, understanding the way water flows through the system is critical.
Seasoned farmers have always made decisions based on years of experience, but natural fluctuations in harvests and a greater concern for the environment has more recently inspired investigation of unfamiliar situations. They’re now looking for better ways to determine how to grow more shellfish faster and how localized nutrient concentration is affected by the presence of the farms themselves. The ISA, with funding from the Irish government, asked BHH to create a computer model that would take these and other concerns into account and serve as a tool for making better decisions in the future.
![]() This FieldView visualization simulates optimized seeding density for maximum meat yield (tons per hectare) using bottom culture technique. Mussels are dropped directly onto the floor of the ocean inlet, grow to harvest size, and are picked up with dredges. Inlet entrance is at right; tide flows in and out from right to left and back. |
A Systematic Approach to Growth Analysis
John Richardson, president and principal engineer at BHH, and Carter Newell, a marine biologist and president of Pemaquid Mussel Farms in Maine, have worked with computer modeling since the 1980s. Clients of theirs include commercial growers’ groups in Virginia, Connecticut, Washington, and British Columbia.
The duo’s first project involved modeling bottom-culture farming techniques. The two analysts used a numerical modeling approach to identify culture sites that would support growth of the greatest number of shellfish. Since this type of analysis involved working with the combined parameters of both food consumption and fluid flow, they needed an advanced computing capability that was easy to use.
Computational fluid dynamics (CFD) computer programs are designed to simulate fluid flow in three dimensions and are sold by at least a dozen commercial companies. All such codes can predict the movement of particulate (nutrient) matter in the water, but calculating the interaction of the food particles with the shellfish, and doing so efficiently, narrowed the options to FLOW-3D from Flow Science of Santa Fe, NM. This software’s ability to model flows through porous media, as well as its easily customizable structure, made it the cost-effective choice.
Modeling Shellfish: Water Interactions
Richardson, a mechanical engineer with 15-years of FLOW-3D modeling experience, designed a system where a menu-screen would prompt the user to input such field data as number of longlines, their length and spacing, drop-line spacing, rope length, rope diameter, shellfish density, particulate availability, and water flow speed. Once a given set of inputs was defined, FLOW-3D would calculate mean values for food concentration in micrograms per liter at any location inside of the computed region, as well as the change in water-flow speed as the mussels grew or more were added to the production site. These simulated results provided a framework for evaluating alternative farm layouts, which could then be run with a revised set of input parameters. The results are visualized using FieldView software from Intelligent Light.
Behind the menu-screens lay the processing power of FLOW-3D. Of particular interest was the approach used to identify obstacles in computed regions. FLOW-3D uses a method known as Fractional Area/Volume Obstacle Representation or FAVOR for short. Unlike other, less flexible CFD programs, this software performs calculations in a structured grid where cells are partially blocked or completely blocked depending on the location of obstacles in the computed region. This practical approach to grid generation gives the user the control to model flows with a high degree of detail in some regions while modeling flows more coarsely in others (thus minimizing overall calculation times).
![]() This FLOW-3D simulation was created to optimize longline raft placement in a mussel fishing ground. The flow patterns are simulated to measure speed of the current at a particular location. Speed changes occur as a result of different rope spacings (flow rate colored by speed; red is fastest). |
Richardson defined areas occupied by shellfish as porous obstacles with the porosity of each based on the number of shellfish present. As a calculation proceeded, the porosities of each region were changed based on the amount of locally consumed food. In addition, the availability of food downstream was reduced by the amount of food already consumed. Accounting for these consumption effects forms an important factor in the design of shellfish aquaculture farm layouts.
Once an obstacle is located within a modeled region, FLOW-3D lets users give it multiple properties—not only can you make the object porous, you can let it move with the water’s flow, and you can even designate thermal properties. It’s these special features that Richardson particularly likes, again contrasting the software’s approach to that of most other fluid-flow packages.
“The FLOW-3D input structure is compact and very flexible,” says Richardson. “With a little creativity, the setups for very unique problems can be constructed, giving you a lot of value and insight for the money.”
According to Richardson, the ability to model flow through porous regions in FLOW-3D is perfect for determining stocking densities that maximize production based on a given set of growing conditions. He found it easy to incorporate both porous- and open-flow regions in his models; he noted, too, that the preprocessor automatically remeshes the problem when you want to rerun the model with a different setup. Other CFD programs can require complete manual remeshing of the problem every time an obstacle is moved.
Field Results Versus Simulation Results
Using field data collected by Newell to calibrate FLOW-3D’s performance, Richardson was able to correlate predicted flow speeds inside the farming structures to actual measurements within a 4.5 percent error. Food concentrations were also measured at different locations, and the simulated values showed less than 3.9 percent error. Other factors that were considered, such as rotating and anchoring the longlines to be oblique to tidal currents, were shown not to have a significant effect on the availability of the food supply and therefore not worth a layout change.
![]() This simulated flow around an individual mussel clump on a rope hanging from a longline is accomplished using FLOW-3D from Flow Science. The top view, side view, and end view (from left) are colored by water speed in feet-per-second, and visualized with FieldView software. |
Taken together, the FLOW-3D results showed how farmers could adjust the stocking density and distribution of their shellfish to maximize the productive capacity of their farms. Shellfish growers have subsequently made a number of changes to the size and distribution of growing areas in Killary Harbour and are reaping the rewards of this work. Furthermore, the Sea Fisheries Board (BIM) is making this analysis available to other interested parties in Ireland.
Richardson and Newell are continuing to use their study methods to help shellfish growers increase their production and minimize their impact on the environment in the United States and abroad. Closer to home, the duo is using the analysis tools to improve the production of their own mussel and oyster farms located on the Damariscotta River in Mid-Coast Maine, and in the mouth of the Penobscot River Down East.
According to Richardson, “It is FLOW-3D’s inherent simplicity that lends itself to use in real-world problem solving. Rather than spending large amounts of time pre-processing (i.e., setting problems up), users can spend their time applying the results of their work.”
More Info:
Blue Hill Hydraulics
Contributing Editor Pamela J. Waterman, DE’s simulation expert, is an electrical engineer and freelance technical writer based in Arizona. You can send her e-mail to [email protected].
Subscribe to our FREE magazine,
FREE email newsletters or both!Latest News
About the Author

Pamela Waterman worked as Digital Engineering’s contributing editor for two decades. Contact her via .(JavaScript must be enabled to view this email address).
Follow DE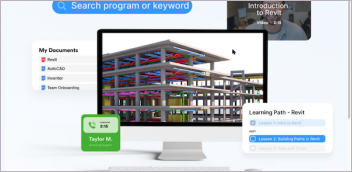
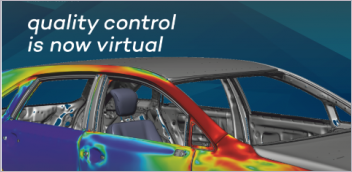
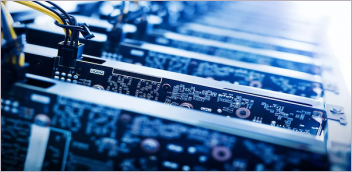
