
Building off lessons learned from the Aurum vehicle, UofM’s Solar Car team is taking a more aggressive systems engineering approach with its latest design. Image courtesy of Michigan Engineering.
Latest News
January 3, 2017
As a physics major and a sophomore at MIT, Veronica LaBelle vowed from the start to take advantage of every opportunity and resource the prestigious university could offer. The roll-up-your-sleeves, can-do spirit of the Solar Car Challenge piqued her interest and LaBelle hopped on board.
Fast forward a year and LaBelle now serves as captain for the MIT SEVT (Solar Electric Vehicle Team) and is gearing up for the world competition in Australia next fall. More importantly, she’s relishing the hands-on learning experience and says the crash course in aerodynamics and lightweighting far exceeds anything she could soak up in the classroom.
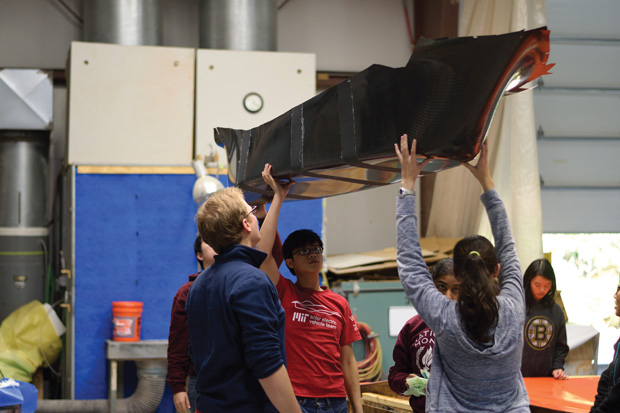
“It’s been an invaluable experience compared to the classroom where you learn about a concept, work out an equation or do a lab,” she explains. “Getting actual hands-on experience can’t compare to classroom knowledge, and there’s something about being set free with other students to produce a car that actually runs.”
The 2017 Bridgestone World Solar Challenge—the grand prix, if you will, of the student solar car competitions—tasks teams to build an energy efficient solar vehicle that will be raced in October in Australia, on a 3,000 km journey from Darwin to Adelaide. The challenge, an exercise in design and energy management, limits solar car contenders to 5kW hours of stored energy (about 10% of what would be required) and tasks teams to come up with a design that draws the remaining energy from the sun or recovered through the kinetic energy of the vehicle.
The World Solar Challenge, like so many student engineering competitions, not only gives student competitors an opportunity to showcase their design chops and benefit from hands-on learning, it has also become a breeding ground for advances in lightweighting and aerodynamics techniques that have commercial applicability.
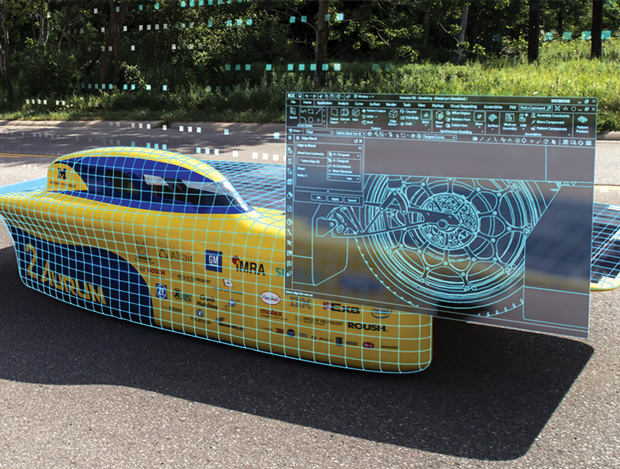
“This is not just a little science project for students to get their head around,” says Paul Lethbridge, student and startup program manager at ANSYS, which sponsors the MIT solar car team and about a dozen or so others globally. “These teams get fully immersed in very complex problems and they are solving them with the highest degree of engineering possible.”
World Solar Challenge Designs in Flux
Next year’s challenge for the class of single-seat solar cars designed for speed has a litany of requirements, some old, some new. For example, the solar car must fit inside a right rectangular prism 5,000 mm long, 2,200 mm wide and 1,600 mm high; the primary source of energy is solar irradiation collected by the car itself; there are numerous safety standards (including rear vision systems) and the solar cars must be able to negotiate a figure eight course in less than 9 seconds per side and less than 18 seconds overall, according to the official guidelines. New to the mix this year are requirements that the cars have four wheels to make them closer to commercial passenger cars, the solar array area has been reduced, and there is a new requirement for a driver-controlled parking brake on all road wheels.
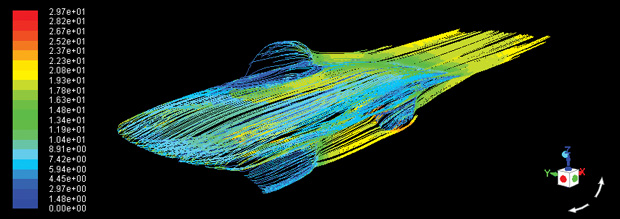
For the MIT team, the new regulations meant a reevaluation of its past generation Arcturus vehicle, which LaBelle says was a three-wheel design and leaned toward being overweight to ensure stability. With Flux, the new vehicle being readied for the 2017 race, lightweighting is a key design objective. It’s being accomplished in three main ways: The team is using SOLIDWORKS finite element analysis (FEA) to determine where it can change materials, from steel to aluminum whenever possible; it’s identifying areas where it can cut back on materials; and the biggest enabler—it’s making increased use of composite materials throughout the vehicle. Specifically, Flux’s new composite monocoque chassis is integrated into the composite lower body and serves as the backbone of the car to reduce weight, LaBelle says.
In addition to liberal use of composites, Flux uses an asymmetric design to position the driver on one side of the car in an effort to create a reduced frontal area that will minimize aerodynamic drag and power consumption, she explains. In addition, the aerodynamics team is iterating designs for the fairings and airfoil-inspired body to maximize laminar flow. Most of the modeling work was done in Rhino 3D, and ANSYS’ Fluent computational fluid dynamics (CFD) was tapped to simulate the shape of the car for optimal aerodynamics.
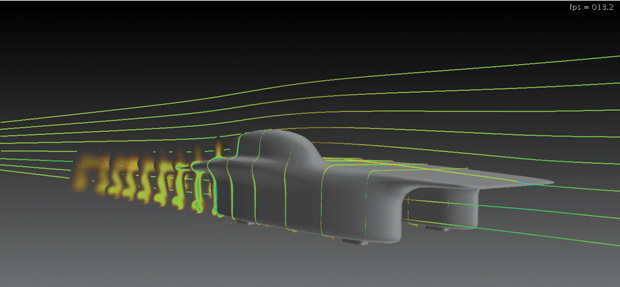
Industry could learn a thing or two from the MIT team’s work in aerodynamics and composites, notes ANSYS’ Lethbridge. “The MIT car has one of the lowest drag coefficients and they have done a lot with lightweighting as well,” he explains. “The use of composite materials is much more prevalent on this vehicle for lightweighting the suspension. Making use of modern materials for suspension components is very challenging because they take a hammering.”
Building a Balanced Car for the World Solar Challenge
The University of Michigan is another long-standing contender in the solar car races, having come in third place a number of times, but never scoring a victory. Building on what it learned with its Aurum vehicle, which competed in the 2015 race, the UofM team went back to the drawing board and decided to emphasize systems engineering tradeoffs as opposed to a straight focus on lightweighting for its next-generation vehicle.
“From the design of Aurum, we discovered that aerodynamics and lightweighting as well as other aspects (solar panel efficiency and battery design) can significantly impact each other,” explains Jiahong Min, a first year graduate student studying mechanical engineering and the lead on aerodynamics. “We are doing more analysis and studying tradeoffs. As a result, the fastest car might not be the lightest, the most aerodynamically efficient or produce the most power from the sun. It would be the car that has the perfect balance between all these aspects and that’s the design we are going for.”
The team uses NX CAD software from Siemens PLM Software to model designs and perform structural analysis. In addition, Altair’s OptiStruct optimization software is employed to help minimize weight while ensuring all the load cases are properly met according to Joshua Agby, lead mechanical engineer and manufacturing director for the UofM Solar Car team. Another big change with the next-generation car is moving simulation and optimization up further in the process as opposed to doing the work on the back end, Agby says.
“In our traditional workflow, we might model a component and then put it into a structural analysis solver to see if it passes the load cases and if it didn’t, we modify it,” he explains. “Now, we use the optimization software to design the part and then check with the structural solver, and nine times out of 10, it’s right. Ultimately, it saves time and you get a better part.”
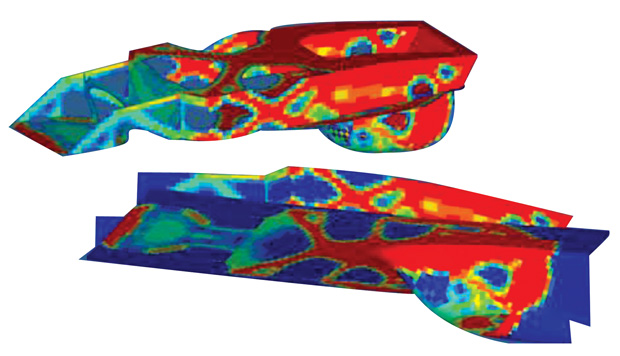
Introducing students to simulation-driven design workflows is one of the main reasons Altair actively sponsors a lot of these student competitions, including the UofM Solar Car team, among others, according to Darius Fadanelli, Altair’s director of Support Operations for the United States. “We want engineers to get comprehensive and accurate simulations so they can dramatically improve products and make better decisions,” he says. “The best place to promote simulation-driven design is with students.” In addition to OptiStruct, the UofM Solar Car team uses a variety of other Altair tools, including HyperMesh and HyperView.
PLM is another enabling tool used first in the Aurum effort and now being carried over the to the next-generation car, Agby says. The team is using Siemens PLM Software’s Teamcenter to help manage and integrate files from the different disciplines, a task it had struggled with previously. PLM is also critical for enabling the systems engineering approach and focus on design tradeoffs, he adds.
Giving student teams exposure to tools like PLM along with getting them to pay serious attention to a design process built around tradeoffs is critical to building skills that will have lasting value over the course of their careers, explains Dave Taylor, global vice president of Marketing at Siemens PLM Software, which is a sponsor of the UofM Solar Car team, along with a team at the University of Leuven in Belgium and Principia College in Illinois.
“Tradeoffs are critical—they might start with ligh weighting as an objective, but find that by reducing the amount of material or using different material, they are suddenly faced with new challenges like a vibration or something breaking,” adds Ravi Shankar, director, global simulation product marketing, at Siemens PLM Software. “None of this can be looked at in isolation, and they need to learn to take a holistic view.”
There’s no doubt the Solar Car team experience has taught Agby that and much more. Getting hands-on experience in FEA simulation is something not covered much in the engineering curriculum, and the lessons on how to collaborate effectively as a multidisciplinary team are invaluable and can’t be replicated in a classroom environment, Agby says.
“Had I not joined the Solar Car team, I’d be a much more ignorant engineer,” says Agby. “I’ve been forced to open up my mind and learn about other disciplines and work with people who may not understand what I’m doing. That’s something you do daily in the workplace and is hard to learn in class.”
More Info
Subscribe to our FREE magazine,
FREE email newsletters or both!Latest News
About the Author
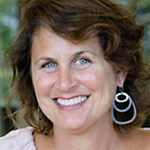
Beth Stackpole is a contributing editor to Digital Engineering. Send e-mail about this article to [email protected].
Follow DE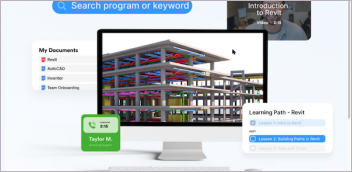
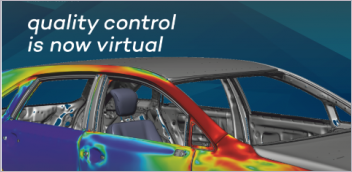
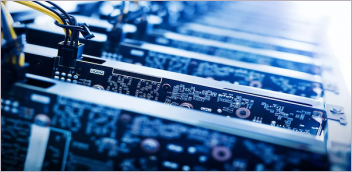
