Latest News
November 1, 2013
By Bernie Rosenthal
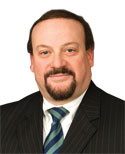
Perhaps the top challenge faced by today’s engine manufacturers is designing high performance internal combustion engines that meet regulatory mandates for reduced levels of greenhouse gases and other toxic emissions. Combustion computational fluid dynamics (CFD) simulation offers design engineers the potential of creating lower emission engines while reducing the millions of dollars spent on physical prototyping and experimentation. However, to ensure that engine simulations can accurately predict real-life fuel emissions, both complex mathematical algorithms that describe the physics and thermodynamic behavior of combustion, and a detailed understanding of different types of engines and the chemical makeup of fuels is required.
Virtual prototyping of engines using CFD allows designers to exploit strategies that vary fuel ignition timings and control other combustion parameters and use simulation results to guide design decisions that can impact engine emissions. However, accurately modeling real fuel behavior requires much more detailed chemistry models than conventional CFD packages can handle.
The Model Fuels Consortium (MFC), an industry-led program, has developed the detailed chemical models required to simulate how fuel behaves during combustion. But, commonly used methods to reduce the complexity of this information to the point that it can be incorporated into traditional CFD simulation approaches result in a substantial loss in predictive accuracy for such impacts as emissions.
This issue is exacerbated by the fact that new, high-efficiency, low-emissions engine designs present technical challenges that are highly influenced by chemistry (e.g., dual-fuel engines, staged spray injections, and premixed charge compression ignition combustion). What proved to be good enough for the development of yesterday’s engines is insufficient for today’s designs.
In addition, the fuel landscape continues to evolve and become more complex as today’s engine specifications demand fuel flexibility (not just gasoline, but various fuel blends with ethanol; and not just diesel, but also biodiesel) while achieving lower emissions.
New engine designs, more complex fuel types, and stricter emissions requirements have meant that combustion simulations need to do a lot more computation—in fact, chemistry calculations can account for as much as 90% of CFD simulation time.
In the past, the typical way designers have dealt with time-to-solution issues was to simply buy more CPUs, or run severely reduced data sets to produce a simulation in a reasonable amount of time. However, neither is a good answer: The first leads to increased capital expense, and the latter limits a designer’s ability to accurately predict emissions and other combustion effects.
Combustion Modeling
Identifying the correct combustion trends through simulation is critical to making good design decisions. Conventional CFD simulations for spark ignition engines are highly mesh-dependent, involving the generation of a computational grid that approximates the dynamics of fuel combustion. These complex interactions require severe mesh refinements and very small cell sizes to resolve flame location and topology. Conventional CFD solutions are also limited in the amount of chemistry detail they can incorporate within a simulation. Accurately modeling real fuel behavior requires more chemistry than traditional CFD approaches can handle while delivering acceptable time-to-solution.
Reaction Design’s FORTÉ CFD Package uses well-established computational techniques, not complex mesh refinements, to create realistic 3D modeling of internal combustion engines. This solution couples proven mathematical techniques and algorithms with detailed chemistry input libraries to simulate spark combustion and predict the effects of operating conditions and fuel variations on engine emissions and particulate matter.
With innovative approaches to relieving the bottleneck in chemistry calculations, predictive engine simulation is now a real tool that can be harnessed for reducing greenhouse gas and toxic pollutants from internal combustion engines.
Bernie Rosenthal is chief executive officer at Reaction Design. Previously, he was the co-founder and senior vice president in charge of worldwide business operations at Tensilica, and has held various executive and management positions at Synopsys, AMCC and TRW. Contact him via reactiondesign.com or [email protected].
Subscribe to our FREE magazine,
FREE email newsletters or both!Latest News
About the Author
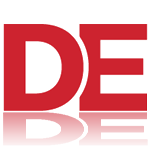
DE’s editors contribute news and new product announcements to Digital Engineering.
Press releases may be sent to them via [email protected].
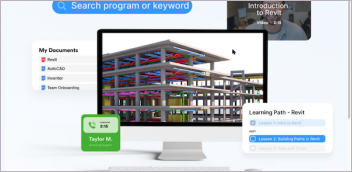
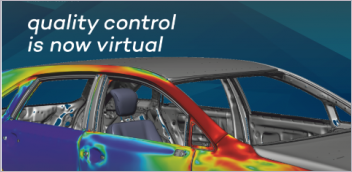
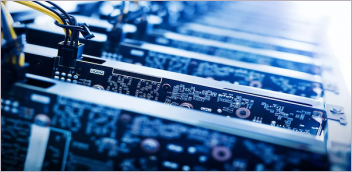
