Creating Carbon Capture Systems
Thanks to an ability to generate lightweight, organic-shaped structures, 3D printing technologies are enabling next-generation systems tasked with greenhouse gas reduction.
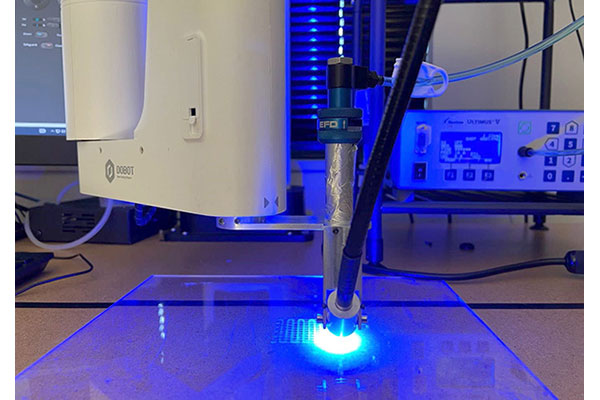
NC State researchers showcase the possibility of making carbon dioxide capture filters using 3D printing. Image courtesy of Sen Zhang and Jialong Shen, NC State.
February 5, 2024
As additive manufacturing (AM) matures, the technology is at the forefront of bringing diverse innovations to life. It ushers in a new genre of personalized medicine through custom implants and surgical guides. It’s also the driving force behind large-scale structures aimed at creating communities of affordable, ecofriendly housing and providing shelter for extraterrestrial explorers heading for Mars and the moon.
Back on earth, AM is playing a key role in the design and production of carbon capture and storage (CCS) systems, a technique aimed at combating climate change by reducing carbon dioxide (CO2) emissions. These solutions capture and separate the carbon dioxide produced by large-scale industrial processes like power plants or steel and cement production, doing their part to tackle the emissions challenge.
While there are different technologies and processes at play, the main idea behind CCS is to capture and separate CO2 from other gases created in industrial operations that are heavily dependent on fossil fuels. The captured CO2 is then compressed and transported to be stored at some sort of site—most commonly, in saline aquifers, pumped underground or even shuttled to depleted oil and gas reservoirs. There are also efforts underway to repurpose the captured carbon to make revenue-generating products such as plastics, concrete, carbonated drinks and carbon-neutral fuel.
The current crop of CCS projects are storing about 45 million tons of CO2 each year, equivalent to the amount of CO2 emissions created by 10 million passenger cars, according to one MIT explainer on the topic. The Global CCS Institute reports there were dozens of projects moving into development in 2022 with the CO2 capture capacity of all facilities growing to 244 million tons a year, a growth trajectory of 44%, in 2022.
In its Q2 2023 update, the Global CCS Institute recorded 37 operational facilities with 20 under construction, 97 in advanced development and 103 in early development, adding up to a total of 257 facilities pursuing CCS in some capacity.
Why the focus on carbon capture systems as opposed to planting more trees?
“Trees take a long time to be productive and if you’re really aiming to be carbon negative, you need a multilayer solution,” says Scott Green, principal solutions leaders for semiconductor and green technology at 3D Systems. “You need to heavily decarbonate polluters, reduce carbon emissions in general and have sustainable, biological and environmental solutions as well.”
AM in the Spotlight
Organizations building and executing CCS projects are gravitating toward AM technology use for a number of reasons. A CCS, which typically involves chemical process equipment housed in smokestacks or other large structures, is made up of networks of complex parts, including high-surface-area structured cells that aid in the carbon sequestration and filtering process. All of these elements are difficult, if not impossible, to manufacture with conventional production methods. They require parts from multiple suppliers, which creates a labyrinth of logistics and supply chain challenges that can counteract the mission of reducing carbon impacts.
“You can’t make a lot of carbon to pull carbon out—you need to have super-efficient processes,” Green says. “One of the benefits of AM is that it allows for functionally consolidated monolithic parts production so you can take an assembly that normally involves 10 suppliers and break it down into one process stack and single print. That simplifies the supply chain and eliminates any additional carbon footprint.”
AM also excels at producing lattice structures and organic geometries, which are essential to producing filter structures with optimal surface areas and that can accommodate the low pressure drops required for CCS applications. Metal AM technologies, in particular, have a proven track record producing cutting-edge, lightweight micro turbines, heat exchangers, direct air contactors and pump casing designs—all components that have a place in CCS solutions.
“Thermal management, mixing, functional or component consolidation—AM is well known for all of those things,” Green says. “AM can significantly reduce the assembly burden and lead time by printing a solid consolidated part and making use of [computational fluid dynamics (CFD)] or multiphysics simulation to get the preferred, optimal output.”
3D Systems is working with customers on many types of CCS and CCS-related applications. Using AM technologies, the company was able to help customers improve heat exchanger cooling efficiency and reliability by leveraging a unique AM alloy and without requirement for supports, which enabled limitless part complexity, Green says.
3D Systems is also helping companies design and AM-produce lattice structures that serve as the structured packing materials used in CCS applications to filter and collect the carbon as well as components for industrial turbines, impellers and pumps.
In partnership with ION Clean Energy, a 3D Systems team leveraged laser powder bed fusion technology to build ION’s Flue Gas CO2 Capture system consisting of highly efficient, modular cells. 3D Systems’ DMP Flex 350 AM platform, coupled with its 3DXpert design software and LaserForm materials, enabled ION to design a comprehensive, hollow lattice structure that yielded maximum surface area and low pressure drop across the cell.
The additively manufactured device created by the Department of Energy’s Oak Ridge National Laboratory has internal channels through which a cooling fluid can be pumped without coming into contact with the solvent and the gas stream. Image courtesy of Carlos Jones/ORNL, U.S. Department of Energy.
“3DXpert is a very critical part of producing metal parts from a design perspective,” Green says.
AM-Related CCS Exploration
Exploration of the synergies between AM technologies and CCS applications is escalating on the research front as well. For instance, AM is a foundational technology underpinning the 3D CAPS project, an effort to achieve a tenfold productivity increase in increasing flow for two sorbent-based technologies used in CCS and to optimize sorbent shapes using CFD and other modeling tools.
The bigger goal of 3D CAPS is to substantially decrease the size and cost of CCS equipment and help with the trade-off challenges associated with the flow-rate through a reactor, pressure drop and kinetics of the absorption process. The 3D printing process will be orchestrated using digital light-based processing (DLP) equipment.
“3D printing will allow bespoke material configuration solutions not available with current production technologies, allowing maximum interplay between these three competing and complementary elements,” stated a write-up on the effort.
Research efforts are also underway to explore where and how AM technologies can advance novel solutions to aid in carbon capture and reduce overall carbon emissions. In one such example, Oregon State University (OSU) and Sandia National Laboratory were the recipients of a $540,000 grant from the U.S. Department of Energy to explore how to capture carbon dioxide from industrial emissions and sequester it in a mineralized form as part of 3D-printed building materials. Researchers are striving to help the construction industry—which is responsible for generating 13% of global CO2 emissions—reduce its carbon emissions through new 3D printing processes and 3D printable materials.
A depiction of the hydrogel filament/grid fabrication system set-up used by the NC State researchers to 3D print carbon dioxide capture filters. Click here for full-size image. Image courtesy of Sen Zhang and Jialong Shen, NC State.
“In recent years, 3D printing technology for concrete has been gaining popularity in building construction as it is a more sustainable alternative since it reduces both waste and transport costs,” says Pavan Akula, assistant professor of civil engineering in the OSU College of Engineering, and a member of the project. “However, most 3D printing of concrete still relies only on traditional materials that are really carbon intensive.”
Researchers plan to capture CO2 emitted from the lime and cement industries and develop sustainable binders that are capable of storing and mineralizing the captured carbon dioxide into printed building components like walls.
North Carolina State University (NC State) researchers are using 3D printing to create carbon dioxide capture filters. The team is using an enzyme known as carbonic anhydrase (CA) to speed up the CO2 capture in a reactive absorption process and using 3D printing technology to output a hydrogel material capable of holding and stabilizing the CA enzyme.
AM has emerged as a promising technology for integrating physical support fabrication together with enzyme immobilization, says Sen Zhang, a research assistant at NC State.
The NC State researchers are using Direct Ink Writing (DIW) with UV-curable materials, which can be finished at room temperature without heating or an additional chemical cross-linking process, Zhang says. “This approach ensures the safety of the enzymes applied in our process, in contrast to Fused Deposition Modeling, which involves melting materials, our 3D printing method is enzyme-friendly.”
The rapid evolution of AM technology and its widespread availability ensures it can be leveraged for mass production at different locales across the globe, adds Jialong Shen, Ph.D., another study author and research assistant professor of Fiber and Polymer Science in the Department of Textile Engineering, Chemistry & Science at NC State. While there are other ways to manufacture carbon capture filters, AM delivers distinct advantages and is an important part of the mix.
“The technologies are not in direct competition with each other—rather we encourage an all-hands-on-deck approach to tackling the climate crisis,” Shen explains.
At the Department of Energy’s Oak Ridge National Laboratory (ORNL), researchers have designed and 3D printed what they claim is a first-of-its kind aluminum device that enhances the carbon capture process. The multifunctional device was designed to improve efficiency of solvent-based carbon capture processes by removing excess heat while keeping costs low. “When CO2 reacts with the solvent, it produces heat that can diminish the solvent’s CO2 capacity,” says Costas Tsouris, distinguished R&D staff at ORNL. “Reducing this localized temperature spike in the column helps restore the solvent’s capacity and thus the efficiency of CO2 capture.”
The team came up with a novel circular device, which integrates a heat exchanger with a mass-exchanging contactor that fits inside a 1-m-tall by 8-in-wide absorption column consisting of seven commercial stainless steel packing elements.
Close-up of the 3D-printed intensified packaging device created by the Department of Energy’s Oak Ridge National Laboratory shows details of the corrugated plates. Image courtesy of Carlos Jones/Oak Ridge National Laboratory (ORNL), U.S. Department of Energy.
In addition, the 3D-printed intensified packing devices were installed in the middle of the columns in between the commercial packing elements to help reduce the temperature spike. An aluminum alloy was chosen as the material for the 3D-printed intensified packing device because of its excellent printability, high thermal conductivity and structural strength, Tsouris says.
“Through in-situ cooling, the circular aluminum packing devices work to enhance the amount of CO2 that can be transferred from the gas stream to the liquid solvent,” he explains.
The intensified devices also have internal channels through which a cooling fluid can be pumped without coming into contact with the solvent and gas stream—a design that allows the intensified devices to operate as heat exchangers.
“AM makes it possible to have a heat exchanger within the column as part of the packing elements without disturbing the geometry of the column,” Tsouris says. “This approach maximizes the contact surface area between the gas and liquid streams.” The decision to adopt the geometry of the commercial packing elements aided in the development of predictive models used to optimize the design of the intensified packing devices, he adds.
AM fit the bill for ORNL’s efforts as well as many other CCS initiatives because of its ability to handle complex geometries.
“Traditional manufacturing methods are simply inadequate for this task because of the complexity of the cooling channels,” Tsouris says. “The advantages of AM are precision, materials selection, the capability to manipulate the surface roughness and functionality and the heat transfer capability, which helps to optimize the CO2 capture efficiency of the absorber.”
More 3D Systems Coverage
Subscribe to our FREE magazine,
FREE email newsletters or both!About the Author
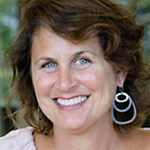
Beth Stackpole is a contributing editor to Digital Engineering. Send e-mail about this article to [email protected].
Follow DE
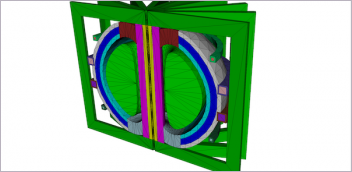
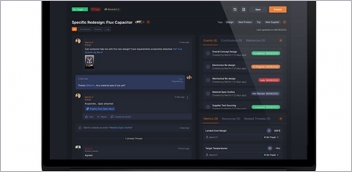
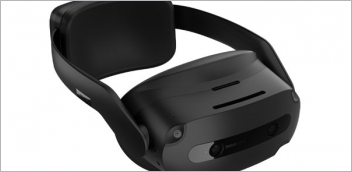