Latest News
May 26, 2007
By Pamela J. Waterman
![]() The electronics housing of this Proba 2 micro-satellite, currently made of aluminum, was used as the basis for a comparative study based on ANSYS software to evaluate the properties of composite materials for a lighter-weight design. (Image courtesy ANSYS and Verhaert Design and Development) |
Far Beyond Golf Clubs
For decades, outdoor enthusiasts have been familiar with the traditional benefits of composite materials, whether trimming the weight of bicycle parts or improving the clout of a golf club. But in more recent years, engineers and designers have identified many more desirable properties, such as thermal conductivity and insulation, and have used FEA tools to help take advantage of these characteristics in a wide range of composite applications.
As far back as the early 1990s, Noran Engineering participated in the Phase I design stage of the X-33 aircraft, a technology demonstrator for NASA’s “next-generation” of reusable space launch vehicles intended to reduce both business and technical risks. One of the vendor teams, led by McDonnell Douglas, used NEiNastran to analyze the performance of a proposed lightweight thermal protection system (TPS) made of a high-temperature ceramic matrix composite called carbon-silicon carbide (C/SiC).
Composites play a critical role in rockets due to their light weight, and are used in two general categories. For the primary and secondary structures, as well as integral load-carrying fuel tanks and non-integral tanks — all relatively “cool” surfaces — ordinary organic composites such as graphite-epoxy are sufficient. However, “hot” areas such as the leading edge of the space shuttle or the overall TPS structure require the thermal properties of the more exotic C/SiC composites.
McDonnell Douglas successfully demonstrated that NEiNastran provided accurate structural and thermal analysis results for a C/SiC system encapsulated with fibrous insulation and laminated to an external metal layer. (The X-33 program itself was later awarded to a Lockheed-Martin team, then subsequently lost its funding and was cancelled.) More recently, Scaled Composites, the aerospace composites development company that won Burt Rutan’s SpaceShipOne contract, chose NEiNastran software as part of its analysis tool portfolio.
![]() General stress analysis of initial X-33 design, as an example of a reusable NASA launch vehicle with composite material elements. (Image courtesy Noran Engineering, Inc.) |
Less Weight, More Challenging
It’s hard to imagine an application where weight matters more than in the payload of a rocket. Componeering, an analysis company in Helsinki, Finland, relied on the advanced analysis capabilities of ANSYS software to create a laminated composite housing material that would give them the comparable thermal and mechanical behavior of the original aluminum while cutting back on overall mass.
The project involved a low-orbiting microsatellite, generally much smaller than a telecommunications satellite. Componeering needed to determine the thermal balance, structural integrity and resonant frequencies throughout the tight spaces, without applying extreme simplifications. The designers evaluated material selection, number of layers, layer orientations, and stacking sequence for a design that embedded a layer of tungsten foil inside a carbon-fiber-reinforced plastic (CFRP) laminate.
Engineers studied laminate through-the-thickness direction and modeled the mechanical interfaces and adhesively bonded joints, including materials, surface roughness, and contact pressure. They performed both conduction and radiative heat-transfer analyses, used that information to directly analyze structural performance, and verified the results through vacuum-chamber testing of a prototype.
The designers then exported the results to Componeering’s own ESAComp software for postprocessing to generate laminate layups and material data, including coefficient of thermal expansion (CTE). The composite design was stiffer, had better thermal properties, and offered a 29 percent weight savings over aluminum.
![]() Composite pipe reducing tee (reinforced) developed using COSMOSM. The Finite Element Model, developed using the COSMOS Command Language, enables dimensional parameters, mesh density, and materials to be quickly changed to assess a wide range of size configurations. (Image courtesy of Grantec Engineering Consultants, Inc.) | ![]() This composite multibranch pipe manifold section model was developed using COSMOSM. Five discrete composite materials are defined through the pipe walls. Input parameters including geometry can be quickly changed to regenerate an FE model to assess various configurations. (Image courtesy of Grantec Engineering Consultants, Inc.) |
Stronger than Steel?
ESG Engineering, a design and analysis company in Tempe, Arizona, wanted to get ahead of the curve demonstrating their expertise in composite analysis to client companies in the aerospace and automotive worlds. It performed its own case-study analysis on a typical vehicle side door designed to compare both performance and cost factors for parts made of conventional stamped steel versus those of fiber-filled composites.
The original 3D CAD door design consisted of inner and outer steel panels (each approximately 0.7mm thick), an intrusion bar, and a number of local reinforcements in the hinge and latch areas. Total assembly count: nine parts. For the alternate design, the engineers evaluated a simple assembly of just two glass-fiber/polyamide composite shells (each 2.65mm thick), with local reinforcements in the inner shell and no intrusion bar.
The project was based on the following three design requirements: the geometry of the original door was left unchanged; composite rupture at smaller deformation levels was allowed as long as the desired strength and energy absorption was achieved; and impact testing required ramming a 12-in. diameter cylinder sideways into the panels to create a static deformation at various depths of intrusion per standard automotive testing.
![]() Stress analysis of proposed advanced thermal protection system for a vehicle such as the X-33. Designed of composite materials (improved carbon fiber protected by silicon-carbon matrix) that would eliminate the current practice of recoating Space Shuttle tiles for waterproofing after each flight. (Image courtesy Noran Engineering, Inc.) |
Serious About Safety
Engine maintenance can account for up to 40 percent of the total lifetime support costs for military aircraft. Much of that comes from replacing parts early as a preventive safety measure, even though they have experienced relatively low numbers of stress cycles. With more and more composites in critical subsystems, it’s important to predict not only total lifetime, but actual life based on the projected severity of small failures. The Royal Australian Air Force turned to the Australian Defence Organization to investigate such probabilities. The group used ABAQUS analysis software from Simulia to predict just where the problems would occur, and then identified the risk involved in crack growth over time.
ABAQUS customers such as Boeing know that this software has long been capable of modeling nonlinear damage and fractures in composites. Its latest revision is also capable of predicting the durability of the adhesives used in composite laminate manufacturing as well as final part assembly. While traditional aircraft used riveted metal sheets (and every drilled hole compromised strength), designs with today’s composites offer equal or superior performance when properly analyzed and assembled. (See sidebar, “ABAQUS V6.7 Unifies FEA with Pre- and Postprocessing.”)
ABAQUS V6.7 Unifies FEA with Composite Pre- and Postprocessing In May, Simulia released ABAQUS V6.7 with enhancements that let designers, engineers, and manufacturing staff the capability model the entire composite design process. Though always capable of analyzing composites, V6.7 now lets users start with the geometry or mesh, incorporate material properties, run the design through the ABAQUS solvers, and directly produce draping and fiber-orientation calculations. Greg Brown, Simulia Product Manager for CAE, notes, “There are lots of niche tools out there, but the real advantage of V6.7 is incorporating ]the information] into a general nonlinear FEA program, with all pre- and postprocessing included.” The unified approach considers such factors as contact and plasticity as well as fastening and bonding techniques for calculating durability in parts made from carbon fiber-reinforced plastics or uni- or bidirectional fabrics. For example, users can take a ply-stacking design generated in CATIA, analyze the linear and non-linear performance in ABAQUS, modify the stack parameters, and export the data back to CATIA in a closed-loop sequence. The process incorporates a plug-in of Advanced Fiber Modeler and Composites Link software from Simulayt.—PW |
When you’ve been successfully transferring corrosive fluids through stainless-steel power-plant piping for years, switching to fiber reinforced plastic (FRP) pipes simply to trim costs isn’t enough. Proving that FRP will do the job equally well was the task taken on by analyst Richard Grant of Grantec Engineering Consultants. A long-time user of COSMOS analysis tools from SolidWorks Corp., he finds the flexibility of the COSMOSM command language gives him the speed he needs to analyze the complexities of composite structural behavior.
The piping design in question had a thin liner surrounded by four additional layers that could vary in their individual designs. By scripting an analysis sequence, Grant could quickly change parameters like the internal diameter (ID) of the main pipe, the IDs of the branches, the thickness of layers, and any material properties to evaluate the effect of reinforcements around nozzle openings. Automatic remeshing made it easy to do multiple studies of pipe diameters ranging from 12 in. to 48 in. while comparing structural results to ASME code requirements.
Grantec Engineering also has years of experience analyzing the structure of large ships, offshore structures, and submerged equipment. Grant says that COSMOSM gives him the ability to readily handle designs with both complex geometries and intense loading by the forces of ocean waves or seismic shocks.
High-Flying Ideas
UGS has had composite analysis capabilities for years in both its NX and Femap lines, as evidenced by the success of the design program for Adam Aircraft’s A500, a pressurized twin-engine aircraft (with both engines mounted along the centerline) featuring carbon-fiber composite (CFC) airframe construction. When Adam Aircraft decided on a CFC skin in the late 1990s, the idea seemed unusual to the conservative general aviation crowd; later, Boeing’s decision to use composites for its 787 Dreamliner made it seem obviously the right choice — just ahead of its time.
The Colorado company chose UGS software because of the ease of interoperability between NX (which enabled surfacing and analysis functions of the sleek composite design) and Solid Edge (whose modeling capabilities generated parts and molds). By using products built on the Parasolid geometric kernel, no time or effort was wasted with data translation. The A500 was FAA certified in 2005, and the company now has back orders for about 70 planes.
Many Players, Many Angles
Knowledge and resources for making the most efficient use of composites also come from users across the manufacturing spectrum. Software such as FiberSIM from Vistagy captures the nuances of the actual composite lay-up approach to help manufacturing teams translate a CAD design into real parts more rapidly, efficiently, and accurately. And suppliers such as Specialty Materials (formerly part of Textron Systems) deliver the real goods with such offerings as titanium metal matrix composite (MMC) preimpregnated sheets.
![]() The Adam Aircraft A500 is a pressurized centerline-mounted twin-engine plane with a carbon-fiber composite skin designed with NX and Solid Edge software from UGS. (Image courtesy UGS) |
Just remember, for technology that seems heavy on verbiage, it’s actually very light stuff, and for many applications, it’s the right stuff, too.
More Information Altair Engineering, Inc. ANSYS Componeering Inc. COSMOS, the Analysis ESG Engineering Grantec Engineering Consultants, Inc. Livermore Software Technology Corporation Noran Engineering Inc. Simulayt Ltd. Simulia, Inc. /Abaqus UGS/Siemens Vistagy, Inc. | Resources for the Hardware Side of the Composite World Whether you’re looking at machines or materials, check these out: W.L. Gore & Associates gore.com Specialty Materials, Inc. specmaterials.com Yokohama Aerospace America, Inc. yaainc.com |
Contributing Editor Pamela J. Waterman is an electrical engineer and freelance technical writer based in Arizona. You can contact her about this article via e-mail here.
Subscribe to our FREE magazine,
FREE email newsletters or both!Latest News
About the Author

Pamela Waterman worked as Digital Engineering’s contributing editor for two decades. Contact her via .(JavaScript must be enabled to view this email address).
Follow DE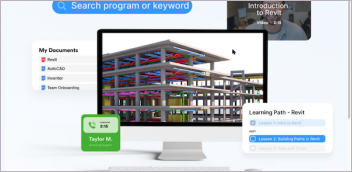
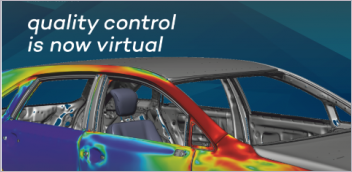
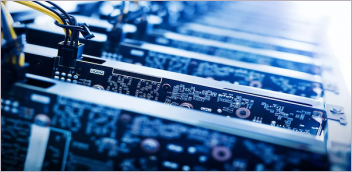
