Latest News
September 1, 2012
By Antoni Drys and Stefano Mascetti
When it comes to virtual modeling, most modern simulation tools already operate at near state-of-the-art. What often limits their use is the uncertainty of a specific, empirical tuning parameter that requires a user to make a best guess. This, in turn, introduces a new source of error—setting up the question, how can you know your choices are well suited to your application?
![]() |
Figure 1: Example of FLOW-3D thermal die cycling simulation, taking into account cooling channel structure. |
Although getting effective results from computer simulations always depends on the quality of the inputs, process simulation applied to the world of metal casting presents particular challenges. Nemak Poland in Bielsko-Biala, Poland, faces these challenges daily as it manufactures aluminum-casting products such as cylinder heads and engine blocks for the automotive industry. The company uses FLOW-3D computational fluid dynamics (CFD) simulation software from Flow Science to model its high-pressure die-casting processes, and is continually working to fine-tune the real-world values it uses for simulation input parameters. Capturing the variations across a complete thermal mold (die) cycle significantly increases the simulation’s accuracy, which in turn yields more realistic temperature and heat transfer properties to guide the choice of equipment settings throughout filling and solidification. (See Figure 1.)
Fine-tuning Process Settings
The different phases of a casting’s thermal cycle involve complex physics, because the speed of the fluid is always changing as it proceeds through each section of the die. Conduction and convection are both present (at comparable magnitudes) in the molten metal, complicating the determination of liquid-to-metal heat transfer coefficients (HTCs). Moreover, it is no simple matter to subdivide the production cycle into well-defined steps to come up with average properties for these effects. Nemak process engineers turned to Sigma Technology to help them zero in on the best operating parameters for setting up their equipment based on the 3D simulations.
![]() |
Figure 2: HTC optimization loop, performed with FLOW-3D simulation software and IOSO optimization software. |
FLOW-3D’s CFD software can model the free-surface flows encountered while filling high-pressure dies, generating results across the entire casting cycle. By tapping into Sigma Technology’s indirect optimization on the basis of self-organization (IOSO) optimization software, now directly integrated with FLOW-3D, Nemak could evaluate possible HTC combinations and feed them back for additional CFD calculations. This closed-loop strategy allowed the engineers to automatically determine new HTCs and manage repeated simulations until the values of theoretical and measured temperatures closely matched. In addition, plans for future part runs could use those same, more accurate HTCs for any successive simulations and new operating profiles (see Figure 2).
Characterizing the Die Process
HTCs are notoriously difficult to determine with high precision. Typically, engineers use coefficient values taken from standard literature references, based on general material properties such as fluid velocities, viscosity, the condition of the heating surfaces and the magnitude of the thermal differential. Nemak started the improvement project by focusing on the thermal behavior of an actual, aluminum alloy (A380) high-pressure die-cast engine block (approximately 200x170x300mm) throughout its production cycle in a die made of tool steel (1.2343).
One full thermal cycle includes all the steps for producing one cast part. This cycle can be divided into homogeneous sub-steps that are performed sequentially; the more sub-steps identified, the more accurate the corresponding simulation results. For this experiment, the thermal cycle was divided into five different segments, each mirrored in the FLOW-3D software model. These sub-steps comprised:
1. metal filling of the die via the shot hole;
2. part solidification and removal;
3. lubrication of the die with a water/oil mixture;
4. blowing out the die cavity to remove excess lubricant moisture; and
5. closing the sections of the die for the next cast.
![]() |
Figure 3: FLOW-3D simulation software run showing high-pressure die-casting temperature vs. time. The regime temperature pattern per cast becomes stable after the initial casting cycles. |
Starting with the die at room temperature, three test parts were cast and discarded—for the sole purpose of allowing the molten metal to bring the tool steel die to its nominal, near-steady operating temperature, called the regime temperature. From then on, because each complete part-production cycle only took 95 seconds, the die never had the chance to cool back to room temperature (see Figure 3).
To capture actual temperature readings, Nemak’s quality-control thermo-cameras were positioned to take thermal images of the die at different times and different locations. Images were observed over the course of a month, and average values were used for comparison to the simulated values at the end of each processing step.
Simulation with a Power Boost
A CAD model of the sample part was loaded into FLOW-3D. Because cooling channels play an active role in the die-casting process, FLOW-3D’s ability to model both the part and the function of the channel structure helped produce results with high fidelity to the real-world Nemak system. For the actual analysis, each of the five sub-steps was loaded with the inputs of the average temperature of the surrounding liquid, the duration in seconds and an initially chosen HTC.
A single FLOW-3D simulation consists of running several cycles of the die through all the sub-steps until the calculations converge to identify the regime (base) temperature. The software automatically brings the die in contact with the different fluid medias (molten metal, lubricant, air, etc.) for the prescribed duration, simulating the thermal exchange. Those values are then compared to those recorded through the thermo-camera. No fluid dynamics simulation is involved at this stage, only a simple thermal simulation, which is much faster.
Use of standard heat-transfer values in the CFD simulation initially produced calculated temperature values that differed by up to 50 Celsius degrees from the measured thermo-camera values. In addition, the simulated distribution pattern across the die did not correlate well. Clearly, the imperfect input data demanded another level of effort to generate more accurate results. The team brought in the IOSO tool to rapidly refine the simulations and zero in on optimized HTCs.
![]() |
Figure 4: Comparison between initial and final FLOW-3D die-casting simulation solutions and thermo-camera values, after optimization of HTCs with IOSO software from Sigma Technology. |
Optimization of complex physical processes, such as those found in CFD analyses, is historically a non-trivial problem. IOSO software solves it by integrating mathematical models, engineering prototypes and exploration methods inside a unified optimization environment based on direct stochastic formulation. Taking a statistical approach to evaluating response surfaces at each iteration, the IOSO process is particularly fast because it approximates the functions of many variables.
Integrated with the FLOW-3D user interface, IOSO directs multiple simulation runs. It needs only to specify the variables to change, the output to analyze and the mathematical goal(s), called the objective function(s). Nemak set up five HTC variables (one for each step of the cycle), each with an upper and lower constraint, plus a single objective function. IOSO called FLOW-3D automatically, analyzing the results, proposing new values for the HTCs and rerunning the casting simulation again until reaching satisfactory results (see Figure 4).
A Simulation Team Effort
As usually happens with optimization runs, strong improvements were recorded immediately at the beginning of the task. In this case, a 50% reduction of the objective function was reached after only 27 calls. As convergence—a global minimum of the function—is approached, the run-to-run improvement tends to be more moderate. Upon reaching a user-defined accuracy, the software was stopped and the solution analyzed as a candidate for the input producing the best simulation. In this case, although 118 runs were conducted, run No. 49 was accepted as the last run to produce an improvement (see Figure 5).
![]() |
Figure 5: IOSO optimization run minimizing an objective function comparing casting temperature values. |
Compared to the original 50° discrepancy, the final FLOW-3D casting simulation based on optimized HTC values exhibited a better match to real-world temperatures. Differences were typically only 2 to 4°C, with a maximum deviation of 15°C at a single probe-point; overall temperature distribution was also greatly improved. Moreover, the optimized HTCs were within a reasonable range for this particular material and process, adding to their usefulness. These values could now be used as a good starting point for other accurate filling and solidification simulations for 3D models of other parts to be cast using the same machine and alloy.
A subsequent, more critical analysis showed that for some optimal solutions, the newly calculated coefficients lie exactly on the borders of the fixed constraints. This situation may imply that some of the chosen constraints were too limiting, and that even further improvements in the optimal HTC solution set may be identified if the constraints are set more loosely.
Simulation: A Valuable Step
FLOW-3D performs simulations on real 3D geometry, and takes into account possible heat transfer mechanisms—conduction, convection and, if desired, radiation. Yet, because it employs efficient algorithms to mesh and solve complex fluid simulations, each IOSO-directed, autonomous FLOW-3D iteration took less than 30 minutes on a typical desktop computer. Hence, this optimization job took 12 hours to reach near-final (convergence) values without the need for high-performance computing (HPC) machines.
Taken together with the potential improvement possible with relaxed HTC value constraints and further developments in the objective function, Nemak believes even stronger correlations between casting process simulations and real-world performance can be made.
Antoni Drys is a virtual simulation expert in the Product Development Centre of Nemak Poland. Stefano Mascetti is with XC Engineering Srl. Send e-mail about this article to [email protected].
More Info
Flow Science Inc.
Nemak Poland
Sigma Technology
XC Engineering Srl
Subscribe to our FREE magazine,
FREE email newsletters or both!Latest News
About the Author
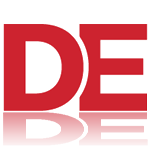
DE’s editors contribute news and new product announcements to Digital Engineering.
Press releases may be sent to them via [email protected].
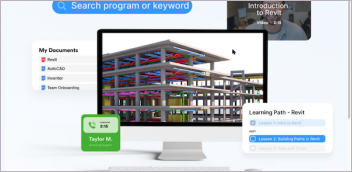
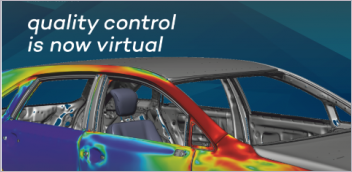
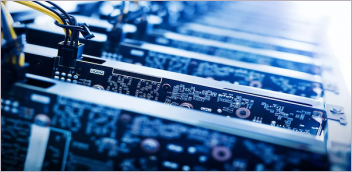
