Additive Manufacturing: A Body of Work
From sockets to instruments, AM is thriving in the biomedical field.
Latest News
April 1, 2013
![]() David Sengeh, a doctoral student at the MIT Media Lab, used SolidWorks and MathWorks MATLAB to design and virtually test the prosthetic sockets to improve their comfort for amputees. |
![]() Sengeh used the Objet Connex 500 3D printer to create sockets that are customized for each patient based on an MRI scan. The printer’s ability to work with multiple materials with different stiffness and flexibility increases the socket’s comfort. |
Additive manufacturing (AM) technology has branched out of its engineering and manufacturing origins into the biomedical field. Today, 3D printers are printing out surgical instruments and prosthetics; in the future, if technological and regulatory hurdles can be overcome, they could even be churning out human tissues and organs. What follow are two very different examples of the state of this art.
David Sengeh: Helping His Homeland
In Sierra Leone, the scars of the civil war that lasted from 1991 to 2002 are still on full display among the population. The decade-long conflict claimed approximately 50,000 lives1. During the war, soldiers from the Revolutionary United Front used amputation as a way to terrorize people, leaving an estimated 4,000 to 10,000 Sierra Leoneans with missing limbs. Very few of these survivors have ever heard of the term “additive manufacturing (AM)”; even fewer could have envisioned machines that can literally print prosthetic pieces. But regardless of whether they know about it, they stand to benefit from the research of David Sengeh, a doctoral student at the MIT Media Lab working with 3D printers.
Sengeh discovered that getting prosthetics made for the Sierra Leoneans was easier than convincing them to wear them. “After speaking to them, I realized that they didn’t want to wear ]the prosthetics] because they’re uncomfortable,” he recalls.
Sengeh says he believes the biggest obstacle to comfort is the socket that joins the artificial limb to the body. Extended use of a prosthetic invariably puts pressure, tension and friction on the bare stump that rubs against the artificial piece; therefore, if the socket doesn’t adequately address these factors, atrophy sets in, creating discomfort.
Sengeh is looking at 3D printers as a solution. The latest advances in the technology have produced machines that are capable of printing with multiple materials, comprising different tensions and strengths. It could be just what’s needed to create sockets with comfortable fit, each tailored to the individual victim with the right geometric profile and sensitivity.
In addition to his biomedical study, Sengeh has another motivation—a more personal one—to help the survivors of Sierra Leone. He grew up in Bo, the country’s second-largest city. The conflict that left many Sierra Leoneans without arms, legs, noses and ears also robbed Sengeh of two uncles.
An Integrated Approach
In Sierra Leone, prosthetic sockets are typically molded out of polyurethane. It’s an efficient, inexpensive manufacturing method for high-volume production. But the resulting sockets are rigid, which discourages amputees from wearing them for extended periods.
Sengeh’s idea is to produce sockets that are customized to each patient’s anatomy based on an MRI scan. The process allows him to create sockets that perfectly match the patient’s bone protrusion. He uses SolidWorks’ mechanical modeling software to create the geometry, and MathWorks MATLAB to study the impact of stress and pressure. For producing these one-of-a-kind sockets, Sengeh relies on a mixture of 3D printing and traditional manufacturing methods.
The pieces that sit close to the patient’s bare skin and bone are better produced in 3D printing using flexible, rubberlike materials. Stronger pieces that serve as structural supports could be produced using conventional manufacturing methods, like injection molding or machining.
Faculty and students of the MIT Media Lab worked with 3D printing system supplier Objet (the company merged with Stratasys in December 2012). Sengeh has access to an Objet Connex 500 printer. As part of its material repertoire, the company offers MED610, a biocompatible material intended for uses involving “prolonged skin contact of over 30 days and short-term mucosal-membrane contact of up to 24 hours,” according to Stratasys. The company also offers a Tango material family, which has rubberlike flexibility. The use of digital material in Objet systems allows Sengeh to create parts made up of multiple materials with varying stiffness and strength.
“Objet parts are pretty flexible,” notes Sengeh. “We’ve been able to show that they have remarkable reduction in pressure on different parts of the body.”
Ideally, Sengeh says, he would like to be able to print with a wider range of materials to produce parts with “super-thin, super-flexible” structures with different stiffness to mimic human muscles. He hopes AM system suppliers can make that happen.
Empowering Technology
Sengeh gets inspiration from his boss, Dr. Hugh Herr, head of the Biomechatronics Research Group, MIT Media Lab. Herr is a double amputee, the result of a climbing expedition that went awry.
“But he’s not disabled,” Sengeh points out. “He lives his life the way I live mine. His is probably even more exciting, because he still goes mountain climbing, running—things I don’t do.”
Herr is outfitted with computer-controlled bionic limbs, one of the Top 10 inventions for health featured in TIME magazine in 2004.
Socket comfort is important not just for Sierra Leoneans, but for anybody who has lost limbs and must wear prosthetics, Sengeh says: “It’s about changing the paradigm of disability. If the users feel comfortable, they’ll able to take on a wide range of activities They can walk, run, do normal things like the rest.”
![]() Sengeh’s socket mounted on a prosthetic leg. Sengeh hopes the improved comfort will convince more amputees in his homeland, Sierra Leone, to take advantage of prosthetics. |
3D Printing Moves into Production
Tom Weisel, president of Ventura, CA-based Arch Day Design, is also deeply involved in developing medical devices with 3D printers. Weisel and his colleagues rely on a 3D printer from Stratasys to create true-scale prototypes when they develop new devices. They used to rely on 3D-printing service bureaus to print their designs, but the typical three-day turnaround was stalling their design cycles. So they purchased an Objet Desktop unit for in-house use.
“We wanted to cut the three days down to three hours,” Weisel says, noting that now, if the Arch Day design team comes up with an idea in the morning, they could be holding the 3D-printed model by late afternoon.
When Weisel suffered a soft-tissue tear on his shoulder, he was in need of corrective surgery. Because he worked closely with doctors, he had no trouble identifying top talent in the field. In post-operation chitchat, he happened to ask the doctor for the brands of the instruments involved. As it turned out, the Arch Day Design team had designed the stitching system and anchoring device used in the operation.
Print Me an Endoscopic Piece
For Weisel and his team, real-world testing of an idea means trying out 3D-printed surgical instruments and medical devices for their cadaver labs. That’s where they invite doctors to experience the reach, comfort, ergonomics and effectiveness of prototyped devices (say, a new pair of surgical scissors) and collect feedback.
“We have to put something in the doctor’s hands to try out an idea,” Weisel says. “They’re performing cutting, stitching, sewing; they’re manipulating something in a human body.”
For example, in certain operations, the doctor needs to pound on the instrument with a hammer. It’s a process that a virtual CAD model cannot satisfy—only a tangible physical prototype would do. In strength, the 3D-printed prototypes are not an exact match to the finished metal pieces, Weisel notes, but with a careful choice of material, the printed parts offer a good representation in stiffness and profile—enough for lab tests.
Arch Day Design chose Objet’s Alaris 30 desktop printer model “because it gives us the accuracy we need at a cost point we can afford,” says Weisel. 3D-printed prototypes for endoscopic tips can be as small as Tic Tac breath mints. Larger pieces could be the size of a coffee cup. The Alaris 30 satisfies the need for both ends of that range, Weisel says.
The firm used to farm out its 3D printing jobs to service bureaus, at a cost of $200 to $2,000 each. The cumulative expense of those jobs over time was significant enough to justify the purchase of the in-house machine, a roughly $35,000 investment. Weisel points out that the time savings—“cutting down a three-day wait to three hours” on each print job—was the real justification for the purchase. Without the usual wait time for outsourced print jobs, the in-house 3D printer allows designers to try out three to five times more iterations, he says.
A Perfect Fit for the Biomedical Field
At the booth of AM manufacturer EOS at the recent Pacific Design & Manufacturing / Medical Design & Manufacturing West Conference (PD&M/MD&M West) in Anaheim, CA, a micro-targeting device currently used in the operating room for deep brain stimulation was on display. Andy Snow, EOS’ regional director for North America, notes that “these are patient-customized devices, FDA-approved for integrated targeting and deep-probe biopsy.”
![]() Arch Day Design, a medical device manufacturer, uses software-based stress analysis to study the strength of a new screw, but the digital model is not sufficient for lab tests. | ![]() The use of an in-house Objet Desktop 3D printer allows Arch Day Design to create physical prototypes in a matter of hours. If the design team has an idea in the morning, the team can be holding the physical mockup by late afternoon. |
AM’s penetration into the biomedical market began with pre-surgical models (used in the planning of surgeries), custom hearing aids, and dental structures. That’s according to Tim Caffrey, associate consultant at Wohlers Associates, who adds that “MRI or CT scan data can go straight to a 3D printer relatively easily. They’re mostly point cloud data, which can then be turned into surface models in software like Geomagic.”
Issues with 3D-Printed TissuesTissue printing seems to be a bit over-hyped at present. Machines that claim to be tissue printers are rather low-grade machines in terms of technology and resolution. There are a couple of groups making some real progress. A key figure is Dr. Dietmar Hutmacher, Queensland University of Technology, Brisbane, Australia. We are still at a very early stage in the technology. Most likely, the initial applications will be applications in joint prosthetics and maxillofacial (fixing problems around the mouth, jaw and neck). No one is actually printing tissue yet. The main issues are the materials that are needed to hydrogel polymers and the fact that they need to be maintained and saturated with water. If you are trying to print tissue, all processes must be at temperatures no greater than body temperature (98.6 ° Fahrenheit) and totally compatible with the well being of cells. That means no toxic chemicals—including many photoinitiators. Also, all materials must be medical grade, which tends to be more expensive and more difficult to process. The biggest challenge for AM system developers to overcome in bio-printing is, everything must be compatible with good manufacturing practice (GMP) regulation, which is a very rigorous quality control, much more severe than ISO 9000. Plus, the software needs to cope with multi-materials and internal architecture. The design of the object must be compatible with a bioreactor to incubate construct before implanting. —Dr. Brian Derby, Professor of Materials Science, Director of Research and Deputy Head of School, School of Materials, University of Manchester, UK |
In conventional manufacturing, the production methods are developed to create hundreds of thousands of identical units. By contrast, biomedical application usually requires one-of-a-kind objects (each pre-surgical model, hearing aid, or dental mold is customized for the individual patient). With its capacity to speedily build 3D profiles based on digital geometry, AM is uniquely positioned to address the needs of biomedical industry in a way that conventional manufacturing cannot.
David Cox, founder and CEO of AM systems distributor Purple Platypus, observes that 3D printers’ biggest advantage is speed.
“You can move from a computer file to an in-hand part, potentially in the space of a few hours, without massive outlays for a mold and/or tooling costs,” he says. “For prosthetics specifically, 3D printing is extremely valuable because it allows the designer the flexibility to customize specific fixtures.
“Multi-material printing that mixes rubber-like flexibility with rigid, plastic-like materials allows engineers to create overmold devices with varying shore values or durometers,” Cox adds. “In an industry that creates medical devices, this is an incredible advancement that helps with ergonomic testing.”
The AM industry has also done much to meet the requirements of biomedical regulatory bodies (the U.S. Food & Drug Administration for example). Wohlers’ Caffrey notes that is especially true in developing materials and processes that are acceptable for producing implants. It elevates the use of AM in biomedical from a prototyping technology to a full-scale production system.
At the PD&M/MD&M West show, also on display in EOS’s booth were titanium hip cups. “There are FDA-approved hip cups on the market that are manufactured using EOS technology,” Snow says.
The titanium powder material used for printing these hip cups, Snow says, “comes from an FDA-approved supply chain.” For medical use with titanium material, EOS recommends the M 280 model, the direct metal laser sintering (DMLS) system. For use with plastic materials, EOS recommends the P 395 midrange model.
“Hundreds of patients in North America are now living with hip cups embedded in their hips that were ‘grown’ via EOS’ DMLS,” Snow adds. “This is not just prototyping technology anymore. It’s now in production use.”
Kenneth Wong is Desktop Engineering’s resident blogger and senior editor. Email him at [email protected] or share your thoughts on this article at deskeng.com/facebook.
References:
1 A Dirty War in West Africa, Lansana Gberie, Hurst 2005.
2 “Disabled in Sierra Leone Make Gains,” Dec. 7, 2011, Global Post, globalpost.com
More Info
3D-Printed Implants Approved by FDA for Bone Replacement
Oxford Performance Materials (OPM) has received FDA approval for its OsteoFab biomedical process. “OsteoFab” is OPM’s brand for additively manufactured medical and implant parts produced from PEKK polymer. OsteoFab uses an EOS P800 and PEKK material to build cranial implants. The implants can be used to repair damage and trauma to the skull, replacing missing bone and integrating with surrounding bone. OPM’s process has already been in use overseas, but this marks the first time the FDA has given approval for AM-created implants to be used in humans in the US. OsteoFab can now be used to treat a variety of patients, including veterans who have suffered severe head trauma. Approval also opens the door for other uses of AM implants. “If you can replace a bony void in someone’s head next to the brain, you have a pretty good platform for filling bony voids elsewhere,” said Scott DeFelice, president of OPM. “We have sought our first approval within cranial implants because the need was most compelling; however, this is just the beginning.” OPM had traditionally sold PEKK as a raw material or in a semi-finished form, but began developing additive manufacturing technologies in 2006. In 2011, OPM established a biomedical compliant manufacturing facility in South Windsor, CT, to support its growing AM business. As an implantable polymer, OPM says PEKK is unique in that is biocompatible, mechanically similar to bone, and radiolucent so as not to interfere with X-Ray equipment. Furthermore, OPM has recently completed testing which it says confirms that the OsteoFab implant surface is, in fact, osteoconductive, so it can be used as a scaffold for new bone growth from the native bone. |
Subscribe to our FREE magazine,
FREE email newsletters or both!Latest News
About the Author
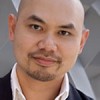
Kenneth Wong is Digital Engineering’s resident blogger and senior editor. Email him at [email protected] or share your thoughts on this article at digitaleng.news/facebook.
Follow DE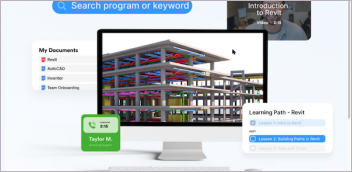
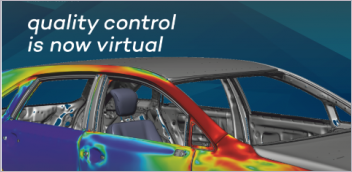
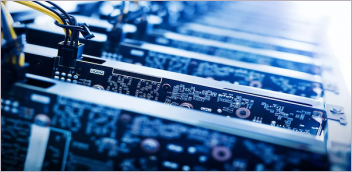
