3D Bioprinting: Moving Beyond 2D Cell Culture
This approach to tissue engineering is getting really exciting (unless you're a robot).
Latest News
August 1, 2013
Less than a decade ago, if you had searched online for “3D bioprinting,” you would have found that the entries would pretty much all point to links at academic institutions, with the exception being pages from EnvisionTEC and nScrypt. Today, a similar search reveals an explosion of work on the subject—encompassing not only corporate-academic partnerships, but also week after week of popular news items explaining the latest progress toward printing functioning organic tissue.
Numerous biological functions, such as developing human tissue, forming human organs, healing wounds and maintaining a stable biological system, depend on the specific architecture of cells and matrix within a given tissue type. The scope of the field in which bioprinting plays a role includes the combination of living cells, with or without supportive scaffoldings, and the technologies that make such creations viable.
In the Beginning
How did this new field of science and engineering progress so far in such a short time? Some of the earliest work went on behind the scenes in the 1990s, when a handful of companies and university researchers started using software-driven dispensers to create carefully assembled cell structures. EnvisionTEC, a German-based company with a strong North American presence, began developing its 3D-Biplotter in 1999; it installed the first commercial unit in 2001. The company is also known for its additive manufacturing (AM) systems based on Digital Light Processing (DLP) projection; scan, spin and selectively photocure (3SP) technology; and liquid resins.
The 3D-Bioplotter system uses a syringe-type dispenser, driven by air pressure, to deposit a wide range of materials that build up and fill/coat well-defined structures. Interchangeable cartridges can be loaded with up to five materials for use in one build. For soft-tissue biofabrication and creating 3D structures that mimic human organs, users can load a dispenser with (but not limited to) living cells, agar, gelatin, chitosan, collagen, alginate or fibrin. For investigations into bone regeneration enhanced by growth-stimulant medications, researchers can combine hydroxyapatite and tricalcium phosphate particles with various resorbable scaffold structures. They can even produce pure ceramic objects.
“The Bioplotter is now in its fourth generation, introduced in 2009,” notes Carlos Carvalho, who is currently lead engineer at EnvisionTEC for process and development. “The accuracy has increased from 50 microns to 1 micron, and reproducibility has also increased, so these systems are now being used in production facilities.”
Carvalho adds that customers are free to choose their materials, many of which are approved by the U.S. Food & Drug Administration (FDA): “The Bioplotter is an open system, so users can control temperature, pressure and speed as desired.”
Build volume for the 3D-Bioplotter is 6x6x5.5 in.; typical structures are created in grid or cylinder form on a baseplate that can be heated or cooled. Customers include Northwestern University and North Carolina State University.
Another relevant company you may not have encountered is nScrypt, a Florida-based resource for 3D conformal printing tools that has also been developing and marketing tissue-engineering (TE) systems since the early 2000s. The TE subseries of nScrypt’s Tabletop dispensing tool is a laboratory-ready system that can build true 3D scaffolds of cell clusters, with varying sizes for precise placement and layering. Users can vary the microporosity depending on materials and printing commands, and dispense materials with viscosities that range from a few centipoise to 1 million centipoise.

nScrypt terms its technology computer-aided biology/computer-aided manufacturing (CAB/CAM), and offers an interesting perspective on how it can support great developments in tissue engineering.
“We can lay down high-definition cells and matrix, or just a matrix itself, and generate a complex three-dimensional structure that very closely mimics the microarchitecture found in the body,” explains Cynthia Smith, an nScrypt bioengineer
Build volume for the Tabletop system is 12x6x4 in. A particularly helpful aspect of the system is that nScrypt’s software interface is designed for bio-oriented users, not computer scientists or traditional CAD experts. The website includes an extensive list of bioprinting materials and their properties.
During this same timeframe, Stuart Williams, Ph.D., was also working on the 3D bioprinting challenge. As founder of the Biomedical Engineering program at the University of Arizona, where he was a professor, he used nScrypt dispenser pumps to build the first version of what he called the BioAssembly Tool, or BAT. With it, Williams 3D-printed one of the earliest examples of a microphysiologic system: a section of lymph node tissue about the size of a dime, deposited into a Petri dish. It was evaluated in vitro and responded to stimuli.
Williams now serves as the executive and scientific director at the Cardiovascular Innovation Institute, a joint collaboration of the University of Louisville and the Jewish Heritage Fund for Excellence. The BAT system, which won an R&D 100 award in 2004, now operates in its next-generation form and is in daily use.
One of Williams’ current projects is working toward creating a total “bioficial” heart built from a patient’s own cells, to serve as a cure for cardiovascular disease. First steps include printing and organizing tissue grown in the lab to build networks of small blood vessels. Thanks to a 2007 grant from the National Institutes of Health (NIH), his group recently printed and implanted some of these vessels and heart tissue into mice.
Discussions of 3D bioprinting must include mention of the work by Thomas Boland, Ph.D., who, in 2004 (then at Clemson University), showed that producing cardiac tissue with off-the-shelf inkjet technology can be improved significantly by using simultaneous scaffold-building and precise cell placement. Previously, a less-efficient process was used to add cells to prefabricated scaffolds.
The Commercial Viewpoint
One 3D bioprinter that’s making a splash in the public eye is the MMX bioprinter from Organovo of San Diego. Founded in 2007, Organovo and its products are the outgrowth of 3D organ-printing research work led by Gabor Forgacs, Ph.D., director of the Frontiers of Integrative Biological Research Program at the University of Missouri, Columbia.
While the hope is to eventually create actual human organs, Organovo’s current work fills an important gap in the development of new drugs. The MMX bioprinter, running with what’s termed the NovoGen process, creates three-dimensional structures that replicate the native form and function of real human tissues. That means biomedical researchers could potentially use the tissue to test drugs or investigate the effect of certain diseases, bridging the gap between in vitro single-cell cultures and larger-scale animal studies.
Michael Renard, executive vice president of Organovo’s commercial operations, explains the process: “We print scaffold-free using biological building blocks (bio-ink spheroids) composed of human cells and/or mixtures of human cells and appropriate proprietary hydrogels. Our first-generation printer has two materials on deck at any one time, and additional materials are switched in during the printing process as needed. Printing is generally conducted at room temperature—although the printer does include temperature control, which is required for some of our proprietary materials.”
The bio-ink spheroids eventually fuse together, rearranging themselves to into appropriately functioning regions, such as fibroblasts migrating to the outside of functioning blood vessel tissue. The resulting tissue can be 20 cell layers thick.
Renard points out that Organovo definitely understands the challenges inherent in dealing with living cells—and so worked with dispenser developer nScrypt, system manufacturer Invetech and cell provider Zenbio to move beyond 2D cell culturing to 3D printing.
“Our bioprinting process incorporates unique features that ensure successful creation of functional tissues, such as low-shear deposition mechanisms and fabrication speeds, that are rapid enough to prevent destruction of the bioprinted tissue due to nutrient or oxygen deprivation,” he explains.
The company has been in the news quite a bit in recent years regarding a number of its research topics, one of which is the combination of three types of liver cells into a three-dimensional structure similar to that found in a human liver. It’s not yet an actual, transplantable liver, but it possesses critical liver functions, including albumin production and enzymatic activities, so the direction is hopeful. Organovo provides its technology on a selective basis to strategic collaborators; its 3D bioprinting technology has already been honored as one of the “50 Best Inventions of 2010” by TIME Magazine.
Worldwide Research

Another major researcher involved in 3D bioprinting is Dr. Anthony Atala, director at the Wake Forest Institute for Regenerative Medicine (part of the Wake Forest Baptist Medical Center, an academic medical center in Winston-Salem, NC).
The Center’s goal is to apply the principles of regenerative medicine to repair or replace diseased tissues and organs. Atala is known to the public for giving several TED talks in 2009 and 2011 discussing growing complete human organs such as a kidney. His group has already created an artificial bladder by coating a laboratory-grown (but non-3D-printed) structure with a patient’s own cells.
Now Atala’s group is working with its own type of 3D bioprinter. It’s an adapted version of ink-jet printing technology to build and study a variety of bioprinted applications, including on-site “printing” of skin for soldiers with life-threatening burns.
Here’s a sampling of other 3D bioprinting efforts currently underway:
- Cornell University—Lawrence Bonassar, Ph.D., is 3D-printing replacement intervertebral spinal discs, with successful results in lab rats. He has also printed collagen/cartilage ears.
- Oxford University—Hagan Bayley, Ph.D, Gabriel Villar and Andrew Heron have constructed materials via computer-control resembling biological tissues that consist of thousands of connected water droplets. The droplets are encapsulated within lipid films, and can carry out the functions of tissues.
- University of California, San Diego—Nanoengineer Shaochen Chen, Ph.D., is using dynamic optical projection stereolithography (DOPsL) to generate microscale 3D blood vessels. The approach uses a computer projection system and micromirrors to direct light onto selected areas of photosensitive biopolymers and cells.
- University of Toyama Facility of Lifescience and Engineering, Japan—In the late 2000s, Makoto Nakamura, Ph.D., used an Epson printer to print cells into bio-tubes similar to blood vessels; he continues to develop this process.
- University of Michigan—At C.S. Mott Children’s Hospital, pediatric ear, nose and throat specialist Dr. Glenn Green and biomedical engineer Scott Hollister, Ph.D., 3D-printed a biodegradable plastic splint to provide a growth-structure support for an incomplete bronchus in a three-month-old boy. At press time, the now-toddler is doing fine.
- Massachusetts Institute of Technology—Linda Griffith, Ph.D., is continuing work begun by Therics using the MIT-patented 3DPrinting process, on microscale engineering of tissues and organs.
- University of Kentucky—Master’s student Amanda Peter Hart worked with bioactive resorbable silica-calcium phosphate nanocomposite, processed using a 3D rapid prototyping technique and sintered at different temperatures to create porous scaffolds. The project produced ulna bones for implant in rabbits.
CAD for Bioprinting
With all this varied activity, it probably makes sense that in December 2012, Organovo partnered with Autodesk Research to explore the integration of Autodesk software capabilities with the tissue fabrication hardware. Their work centers on designing a new user interface. So far, the program is for internal development only, but it demonstrates how this field is attracting mainstream interest.
Contributing Editor Pamela Waterman, DE’s simulation expert, is an electrical engineer and freelance technical writer based in Arizona. You can send her e-mail to [email protected].
Resources:
Journals and magazines for further reading: Biomaterials, Biofabrication, Tissue Engineering and Trends in Biotechnology.
For More Info
Cardiovascular Innovation Institute
Massachusetts Institute of Technology
Subscribe to our FREE magazine,
FREE email newsletters or both!Latest News
About the Author

Pamela Waterman worked as Digital Engineering’s contributing editor for two decades. Contact her via .(JavaScript must be enabled to view this email address).
Follow DE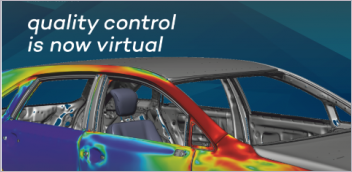
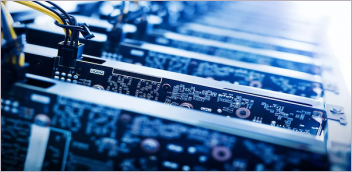

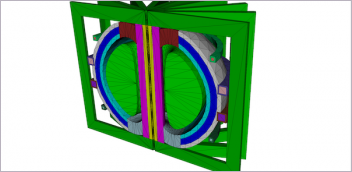